Introduction. Interstellar Probe is a NASA Heliophysics Division mission concept that would leave the solar system on a 50-year prime mission with a primary objective to study the heliosphere and the local interstellar medium (>200 au; Brandt et al., 2020). In order to reach interstellar space, the spacecraft must pass through the trans-Neptunian region, presenting the opportunity to study planets and minor bodies (Brandt et al., 2020). We focus here on the opportunities for exploring trans-Neptunian objects (TNOs) with Interstellar Probe.
TNO Geoscience Investigation. The primary science instrument for TNO flyby geoscience would be a visible/infrared imaging spectrometer to enable studying a wide range of physical, chemical, and geological processes at temperatures <40 K. Reflectance spectroscopy of the infrared region (1-5 μm) is most useful for studying surface composition due to myriad fundamental and overtone absorption bands of volatile (N2, CH4, CO) and non-volatile (H2O, CO2, NH3) ices and refractory organics (e.g., C2H6, tholins). A visible imager would reveal geological, albedo, and color information to investigate surface characteristics and evolution.
Opportunities for dwarf planet exploration. The heliophysics science goals will dictate Interstellar Probe’s trajectory, with a consensus coalescing around leaving through the “waist” of the heliosphere between ecliptic longitudes of 300-330° (Brandt et al., 2020; Runyon et al., 2020). There are an estimated 130 dwarf planets—objects >400 km in diameter—beyond Neptune. In or near this region, between -20° and 20° in heliocentric ecliptic latitude, there are five potential dwarf planets that Interstellar Probe could fly by: 2002 MS4, Quaoar, Gonggong, Pluto, and Ixion (Figure 1).
Potential dwarf planet targets represent a wide range of colors, compositions, and geological processes. Quaoar is dense (~2 g cm-3; Braga-Ribas et al., 2013) and may therefore have retained enough internal heat to remain geologically active and/or support a subsurface ocean (e.g., Shchuko et al., 2014). Gonggong and its satellite Xiangliu show one of the largest color dichotomies among a primary and satellite (Kiss et al., 2019), and Gonggong may be a contact binary (Pál et al., 2016). Little is known about 2002 MS4 due to its current position in a crowded star field, frustrating ground-based observations, but it is large enough for possible differentiation and a surface dominated by H2O ice and the resultant geological features. Ixion has a flat near-IR spectrum (Barkume et al., 2008; Guilbert et al., 2009), meaning it could provide clues to the substrate present beneath the ice on all TNOs. Pluto would benefit from a follow-up 20+ years after New Horizons to reveal surficial and atmospheric changes.
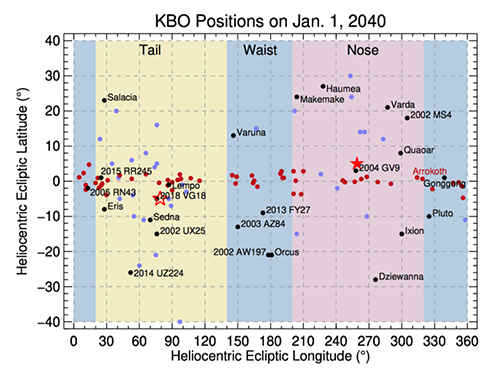
Figure 1. Positions of dwarf planets (black), CCKBOs (red), and extreme TNOs (purple) with respect to the ecliptic on January 1, 2040. Longitudinal regions for the heliospheric nose (pink), tail (yellow), and waist (blue), as well as the heliospheric nose (filled red star) and tail (open red star), are marked. We assumed the trajectory of Interstellar Probe will take it somewhere between ~300-340˚ longitude and -20˚ to 20˚ latitude, opening up the possibility for a flyby of 2002 MS4, Quaoar, Pluto, Ixion, or Gonggong.
Prospects for a secondary target flyby. New Horizons’ flyby of Arrokoth (2014 MU69) in 2019 provided a valuable glimpse into the formation history of planetesimals and the pristine surfaces present beyond Neptune (Stern et al., 2019). The flyby of this small TNO (~35 km) revealed the building blocks of the largest TNO, Pluto, New Horizons’ other target. Interstellar Probe could complement the Arrokoth and Pluto flybys via a serendipitous encounter with a TNO <400 km in diameter, potentially revealing a new class of TNOs never before visited by spacecraft.
We consider the possibility of serendipitous flybys of TNOs <400 km, with the highest probability occurring for a spacecraft trajectory in the ecliptic plane among the low-inclination cold classical Kuiper Belt Objects (CCKBOs). CCKBOs have inclinations ≤5° and semi-major axes between 42 and 47 au (Gladman et al., 2008). From surveys at greater depth, predominantly at low ecliptic latitudes (e.g., OSSOS; Bannister et al., 2016), ~35% of known TNOs fit these criteria (JPL/Horizons Small-Body Database); debiased population assessments suggest that the CCKBOs are the most numerous sub-population of the <100 au region (e.g., Fraser et al., 2014).
An estimated 40,000-60,000 new TNOs to absolute magnitudes >8 will be discovered with the Vera C. Rubin Observatory’s Legacy Survey of Space and Time (LSST; Schwamb et al., 2018). Assuming 45,000, some 16,000 CCKBOs will be known by the early 2030s. Assuming uniform distribution along the ecliptic between 42-47 au, each CCKBO would “occupy” a volume of 0.0875 au2 with a circular radius of ~0.17 au. Defining a reasonable flyby distance of 10-4 au (~15,000 km, comparable to New Horizons’ flyby distance of Pluto), the probability of serendipitously flying-by a particular CCKBO is 3 𝗑 10-5 %. For a trajectory radially outward from the Sun, there would be ~30 opportunities for serendipitous flybys, leading to a 1 in 100,000 chance of making a serendipitous flyby.
This estimate assumes a trajectory near the plane of the ecliptic and Gonggong as the primary target. A more oblique trajectory through the classical belt would improve the chances. For trajectories out of the plane of the ecliptic, the probability of a serendipitous flyby drops precipitously.
References.
Bannister, M.T., et al., 2016. AJ 152, 70.
Barkume, K.M., et al., 2008. AJ 135, 55-67.
Braga-Ribas, F., et al,. 2013. ApJ 773, 26.
Brandt, P. C., et al. (2020). NAS white paper for the Solar & Space Physics Decadal Survey 2023.
Fraser, W.C., et al., 2014. ApJ 782, 100.
Gladman, B., et al., 2008. The Solar System Beyond Neptune. University of Arizona Press, Tucson, 43-57.
Guilbert, A., et al., 2009. Icarus 201, 272-283.
Kiss, C., et al., 2019. Icarus 334, 3-10.
Pál, A., et al., 2016. AJ 151, 117.
Runyon, K.D., et al., 2020. NAS white paper submitted to the Planetary Science decadal survey committees.
Schwamb, M.E., et al., 2018. arXiv:1802.01783.
Shchuko, O.B., et al., 2014. P&SS 104, 147-155.
Stern, S.A., et al., 2015. Science 350, aad1815.
Stern, S.A., et al., 2019. Science 364, aaw9771.