Intoduction. The recent progress in the studies of Pluto is related to the New Horizons flyby, and the solar and stellar occultations at 52-187 nm revealed vertical profiles of N2, CH4, C2H2, C2H4, and C2H6 (Gladstone et al. 2016, Young et al. 2018, Kammer et al. 2020). The ALMA submillimeter spectroscopy (Lellouch et al. 2017) gave a CO profile, abundance of HC14N, and restrictive upper limits to HC15N and HC3N. The photochemical model of Pluto’s atmosphere and ionosphere (Krasnopolsky 2020) reproduces fairly well these data. Recently methylacetylene and atomic hydrogen were detected using UV spectroscopy from New Horizons (Steffl et al. 2020).
Metylacetylene C3H4abundance of 5×1015 cm-2 observed from New Horizons is close to 7.7×1015 cm-2 in our early model (Krasnopolsky and Cruikshank 1999) but exceeds 9×1014 cm-2 in Krasnopolsky (2020). To bring the model into agreement with the observation, rate coefficients of the three key reactions of C3H4 production and removal are changed to the values calculated by Vuitton et al. (2019). The calculated C3H4 abundance is 4.3×1015 cm-2 (Figure 1).

Main reactions of production and loss of C3H4 are shown in Figure 2. Column abundances of some species in the model and its previous version are shown in Table 1 along with production and loss rates, escape and precipitation flows, and chemical lifetimes. The HC3N abundance of 2.3×1013 cm-2 in the model is close to the upper limit of 2×1013 cm-2 (Lellouch et al. 2017). The change in the model increases H2 and C3H8 by factors of 1.3 and reduces H and C4H2 by factors of 1.3, while the other species remain almost unchanged relative Krasnopolsky (2020).
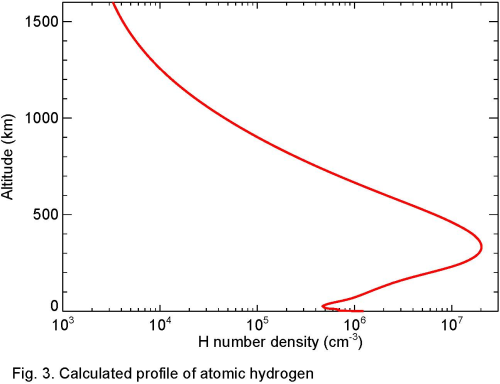
Atomic hydrogen abundance of (7.7±1.7)×1013 cm-2 at τ=1 (490 km) was measured (Steffl et al. 2020) using the observed Lyman-beta emission. This value is 6.9×1013 cm-2 in our model (Fig.3) and 7.3×1013 cm-2 in the previous version (Krasnopolsky 2020).
Reflectivity of 0.17 at 140-185 nm (Steffl et al. 2020) agrees with the HST value of 0.14 at 180-190 nm (Krasnopolsky 2001).
Nitrogen isotope ratio. The observed lower limit HC14N/HC15N > 125 on Pluto (Lellouch et a. 2017) agrees with 14N/15N in the planets, asteroids, comets, and the solar wind. However, it looks puzzling compared to HC14N/HC15N = 60 on Titan that is perfectly explained by predissociation of N2 at 80-100 nm (Liang et al. 2007, Krasnopolsky 2016). Both Pluto and Titan have nitrogen-methane atmospheres with significant similarity in photochemistry.
Major reactions of production and loss of HCN on Pluto are given in Table 2. Our analysis confirms predissociation of N2 at 80-100 nm as the major process of nitrogen isotope fractionation on Pluto. Kinetic isotope effect induced by difference of reduced mass of the reactants is very small, for example, k14/k15 = 1.006 for CH + HCN → CHCN + H. Our calculation gives negligible photo-induced isotope fractionation for HCN. Isotope fractionation in condensation of ammonia is calculated at 1.04 for Pluto’s conditions using measurements by King et al. (1989). This value is adopted for HCN on Pluto.

The observed twofold difference on Pluto is partially caused by diffusive depletion of the heavy isotope in HCN and in predissociation of N2. On Pluto, mean altitudes of HCN and predissociation of N2 are 500 and 860 km, well above the homopause at 96 km. On Titan, the observations of HC14N/HC15N refer to 90-460 km (Vinatier et al. 2007), predissociation happens near 985 km, both below the homopause at 1000 km, and diffusive depletion does not occur. Therefore the observed limit corresponds to 14N/15N > 253 for N2 in the lower atmosphere and 14N/15N > 228 in the upper layers of the N2 ice. These limits reflect the conditions on Pluto in the last two million years. The current loss of N2 is 37.5 g cm-2 Byr-1 primarily to photodestruction. The calculated isotope fractionation factor of 1.96 accounts for formation and condensation of nitriles, diffusive separation, and fractionation in thermal escape. Variations of 14N/15N in the N2 ice are relevant to evolution of the solar EUV, mixing processes in the N2 ice, and possible periods of hydrodynamic escape that are poorly known and not considered here.
References
Gladstone, G.R., et al., 2016. Science 351 (6279), aad8866.
Kammer, J.A., et al., 2020. Astron. J. 159, 26 (9pp).
King, T.V., et al., 1989. Z. Naturforsch. 44a, 359-370.
Krasnopolsky, V.A., 2001. Icarus 153, 277-284.
Krasnopolsky, V.A., 2016. Planet. Space Sci. 134, 61-63.
Krasnopolsky, V.A., 2020. Icarus 335, 113374.
Krasnopolsky, V.A., Cruikshank, D.P., 1999. J. Geophys. Res. 104, 21979–21996.
Lellouch, E., et al., 2017. Icarus 286, 289-307.
Liang, M.C., et al., 2007. Astrophys. J. Lett. 664, L115-L118.
Steffl, A.J., et al., 2020. Astron. J. 159, 274 (12pp).
Vinatier, S., Bezard, B., Nixon, C.A., 2007. Icarus 191, 712-721.
Vuitton, V., Yelle, R.V., Klippenstein, S.J., Hӧrst, S.M., Lavvas, P., 2019. Icarus 324, 120-197 (supplementary material).
Young, L.A., et al., 2018. Icarus 300, 174-199.