The complexity of the phenomena taking place in exoplanet atmospheres is challenging to simulate and choices have to be done to simplify the problem. The choice of using a one dimensional atmospheric model is potentially the most resource-saving one, though when considering effects of transport, a new caveat appear: how to account for such 3D-related processes in 1D ? The answer is: considering all these complex phenomena as a single diffusion process called eddy diffusion (Colegrove et al., 1965; Smith, 1998; Moses et al., 2011; Lavvas & Koskinen, 2017). This is a long known simplification, yet no complete theoretical frameworks exist to accurately assess this parameter and most 1D models assume either a constant eddy diffusion coefficient treated as a fitting parameter (Spiegel et al., 2009), or a profile derived from 3D general circulation models (Parmentier et al., 2013; Zhang & Showman, 2018). Unfortunately, all planets haven’t been studied with such a model and therefore, no accurate eddy profile derived from GCMs can be found for these planets. Moreover, the different ways to derive the eddy via 3D model provide very different results. In this work, we aim to develop a parametrization suitable for gas giant exoplanets and to assess the eddy profile obtained for ten hot-Jupiters.
We developed an eddy profile parametrization based on parameters available in 1D calculations. The parameterization separates the atmosphere in three parts: a convection-driven mixing below the radiative-convective boundary (Ackerman & Marley, 2001), a middle atmosphere eddy diffusion increasing with decreasing density in a √1 behavior (Chamberlain, 1978), and an upper atmosphere above the homopause where we consider a constant eddy diffusion coefficient scaled on Jupiter’s homopause eddy mixing (Koskinen et al., 2010).
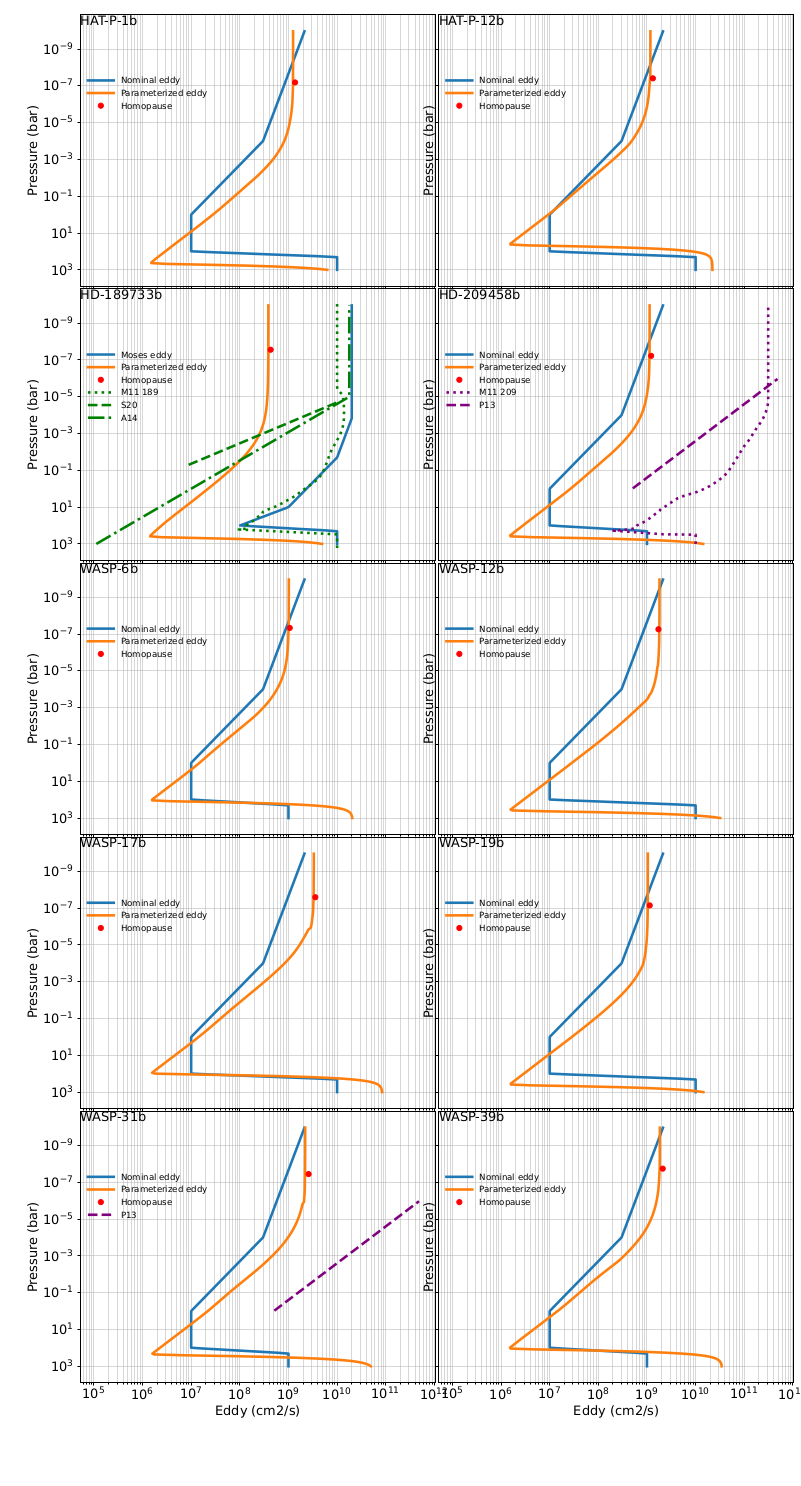
Figure 1: Nominal and parametrized eddy profiles. When available, additional GCM results are shown (M11 for Moses et al. (2011), S20 for Steinrueck et al. (2020), A14 for Agùndez et al. (2014), P13 for Parmentier et al. (2013)).
The eddy profile affects the transport and then the distribution of chemical species and hazes in the atmosphere, thus resulting in modifications of the transit spectra. Therefore, in the purpose of testing this parameterization, we use a self-consistent 1D model that couples haze microphysics (Lavvas & Koskinen, 2017), disequilibrium chemistry (Lavvas et al., 2014) and radiative transfer (Lavvas & Arfaux, 2021). We conducted two sets of calculations: the first with a nominal eddy profile based on the profile derived by Moses et al. (2011) for HD-189733b and downscaled following Parmentier et al. (2013) results, and the second with our parametrization (Fig. 1). The modification of the eddy can bring important modifications of the haze particle distribution with a stronger mixing leading to a decrease of the transport timescale. This produces smaller and more numerous particles as they have less time to coagulate. The changes in the haze particle distribution are impacting the transit spectra. While the spectra of haze-free atmospheres present weak variations from the nominal eddy case, the smaller particles obtained for hazy planets result in a significant decrease of the transit depth (Fig. 2). These planets would therefore require more haze to fit the HST observations. This result is consistent with the larger haze precursors mass fluxes we obtain. Indeed, modifying the eddy leads to important modifications of the chemical composition profiles, notably the haze precursors. The stronger upper atmosphere (usually in the region up to ∼0.01 μbar altitude as shown in Fig. 1) eddy mixing produced by our parametrization enhances HCN and CO abundances, which are considered to be the major contributors to the formation of photochemical hazes. This increases the production of hazes with precursors photolysis mass fluxes multiplied by 5 on average compared to the nominal eddy case.
Figure 2: Transit spectra obtained with the nominal and parametrized eddy profiles. The values in parentheses are the mass fluxes assumed for the haze formation in g.cm−2.s−1.
In conclusion, our parameterization produces in most cases eddy profiles rather close to the nominally used, mainly slightly larger in the upper atmosphere. These new eddy profiles require some slight adjustment of the haze mass flux to fit the observations compared to the mass fluxes derived using the nominal eddy profile. We however note the particular case of HD-189733b (Fig. 1). This planet indeed requires a particular care due to its challenging UV-visible observations (Fig. 2) that imply haze mass flux and eddy diffusion significantly larger than for the other planets.
References
Ackerman, A. S., & Marley, M. S. (2001). The Astrophysical Journal, 556(2), 872–884.
Agùndez, M., Parmentier, V., Venot, O., Hersant, F., & Selsis, F. (2014). Astronomy and Astrophysics, 564, A73.
Chamberlain, J. W. (1978).
Colegrove, F. D., Hanson, W. B., & Johnson, F. S. (1965). Journal of Geophysical Research, 70(19), 4931–4941.
Koskinen, T. T., Cho, J. Y.-K., Achilleos, N., & Aylward, A. D. (2010). The Astrophysical Journal, 722(1), 178–187.
Lavvas, P., & Arfaux, A. (2021). Monthly Notices of the Royal Astronomical Society, 502(4), 5643–5657.
Lavvas, P., & Koskinen, T. (2017). The Astronomical Journal, 847.
Lavvas, P., Koskinen, T., & Yelle, R. V. (2014). arXiv e-prints, (p. arXiv:1410.8102).
Moses, J. I., Visscher, J. J., C.; Fortney, Showman, A. P., Lewis, N. K., Griffith, C. A., Klippenstein, S. J., Shabram, M., Friedson, A. J., Marley, M. S., & Freedman, R. S. (2011). The Astrophysical Journal, 737(1).
Parmentier, V., Showman, A. P., & Lian, Y. (2013). Astronomie and Astrophysics, 558, A91.
Smith, e. a., M. D. (1998).
Spiegel, D. S., Silverio, K., & Burrows, A. (2009). The Astrophysical Journal, 699(2), 1487–1500.
Steinrueck, M. E., Showman, A. P., Lavvas, P., Koskinen, T., Tan, X., & Zhang, X. (2020). arXiv e-prints, (p. arXiv:2011.14022).
Zhang, X., & Showman, A. P. (2018). The Astrophysical Journal, 866(1), 1.