INTRODUCTION
Over the past two decades, ground- and space-based observations have unveiled thousands exoplanets and planetary systems around other stars in our Galaxy. About 5000 exoplanets are currently confirmed, in large part detected as transits by the Kepler and TESS missions. Launched in 2019, PLATO mission represents the first-step characterisation towards the understanding of the structural properties of these planets. Nevertheless, a significant boost for the detection of transiting Earth-analogues around bright stars is expected from the PLATO mission.
However, it's only through remote atmospheric spectroscopy of potentially habitable rocky planets, that one of the main goals of exoplanetary science, the quest for life outside the Solar System, can be tackled. This observational challenge should be partly within reach of the recently launched JWST and the next ground-based astronomical observatory, E-ELT.
To accomplish the demanding task of searching for and deciphering spectral signatures, a thorough and holistic observational and theoretical characterization of carefully selected rocky exoplanets is required.
The selection, among the observationally reachable targets for high-resolution spectroscopy of thin atmospheres, requires habitability studies with climate models.
These simulations will enable the identification of those exoplanets with the largest chance of potentially hosting a surface diffuse life, i.e. with the largest habitability, that must be evaluated over a wide range of mostly unknown conditions.
A considerable effort of modelization that exploits all available observations will be needed in order to assess the global physical characterization of the selected exoplanets, and in particular precisely of their potential surface climate and habitability.
THE MODEL
Here we present EOS-ESTM, a flexible climate model aimed at simulating the surface and atmospheric conditions that characterize habitable planets. The model allows one to perform a fast exploration of the parameter space representative of planetary quantities, including those currently not measurable in rocky exoplanets. EOS-ESTM has been built up starting from ESTM (Vladilo et al. 2013, 2015), a seasonal-latitudinal EBM featuring an advanced treatment of surface and cloud components and a 2D (vertical and latitudinal) treatment of the energy transport.
The main features of the model that we have implemented can be summarised as follows.
Firstly, we have calculated the atmospheric radiative transfer using EOS (Simonetti et al. 2022), a procedure tailored for atmospheres of terrestrial-type planets, based on the opacity calculator HELIOS-K (Grimm & Heng 2015; Grimm et al. 2021) and the radiative transfer code HELIOS (Malik et al. 2017, 2019). Thanks to EOS, the ESTM radiative transfer can be now calculated for a variety of atmospheres with different bulk and greenhouse compositions, illuminated by stars with different SEDs.
Then, we have upgraded the parameterizations that describe the clouds properties. New equations have been introduced for the albedo of the clouds and its dependence on the albedo of the underlying surface. The clouds coverage over ice is now a function of the global planetary ice coverage. A specific treatment for the transmittance and OLR forcing of clouds at very low temperature has been introduced.
Lastly, we have introduced a generalized logistic function to estimate the ice coverage as a function of mean zonal surface temperature. Based on a detailed study of the ice distribution on Earth, the adopted algorithm discriminates between ice over lands and oceans. The albedo and thermal capacity of transitional ice is now estimated using the fractional ice coverage.
RESULTS
With the aim of providing a reference model for studies of habitable planets, we calibrated EOS-ESTM using a large set of Earth satellite and reanalysis data.
The reference Earth model satisfies a variety of diagnostic tests, including mean latitudinal profiles of surface temperature (Figure 1), TOA albedo, OLR and ice coverage.
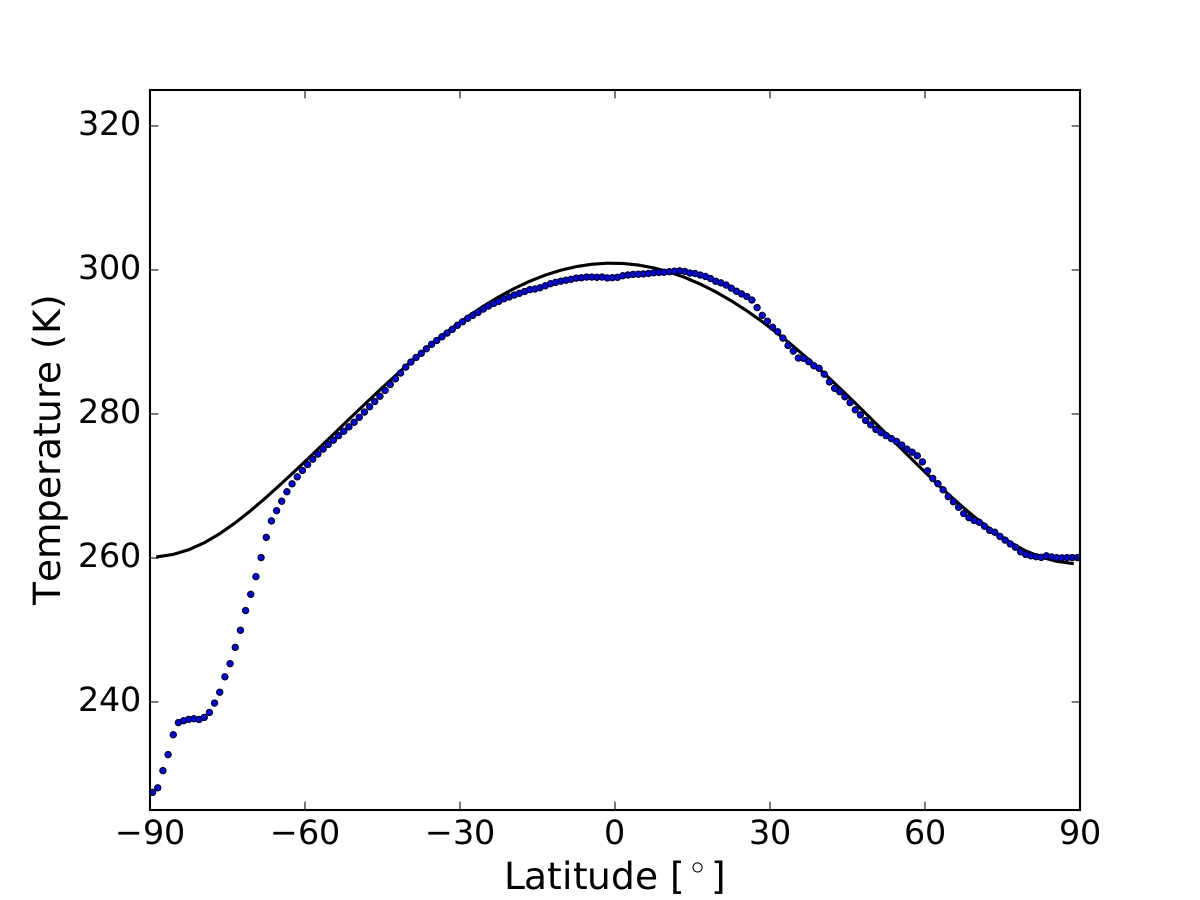
Fig. 1. Mean annual latitude profile of surface temperature predicted by the reference Earth model The temperature profile is compared with ERA5 temperatures averaged in the period 2005-2015 (blue dots).
To test the consistency of EOS-ESTM with previous studies of non-terrestrial climate conditions we performed a series of comparisons with a hierarchy of climate models, varying the levels of insolation (Figure 2), the stellar spectrum and planetary parameters (radius and rotation rate).
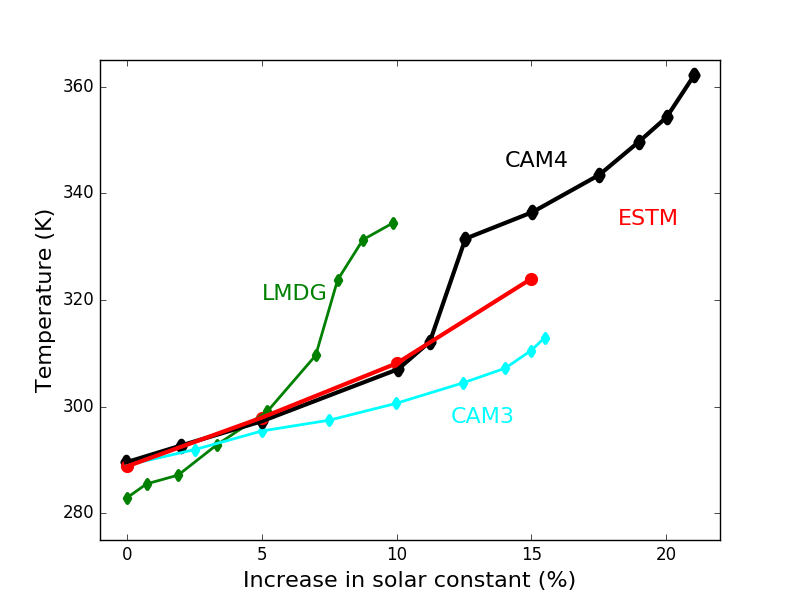
Fig. 2. Comparison of global and annual mean surface temperature obtained from different climate Earth's models by increasing the solar constant. Red, solid line: EOS-ESTM (this work). Black, solid line: 3D model CAM4 (Wolf & Toon, 2015). Cyan, solid line: 3D model CAM3 (Wolf & Toon, 2014). Green, solid line: 3D model by Leconte et al. (2013).
The application of EOS-ESTM to the case of a CO2-dominated atmosphere in maximum greenhouse conditions (Kasting et al. 1993) yields a detailed description of the transition to a snowball state that takes place when the insolation decreases in the proximity of the outer edge of the HZ. Thanks to the flexibility of our model we can explore how this transition develops in different planetary conditions (e.g. rotation rate, Figure 3).
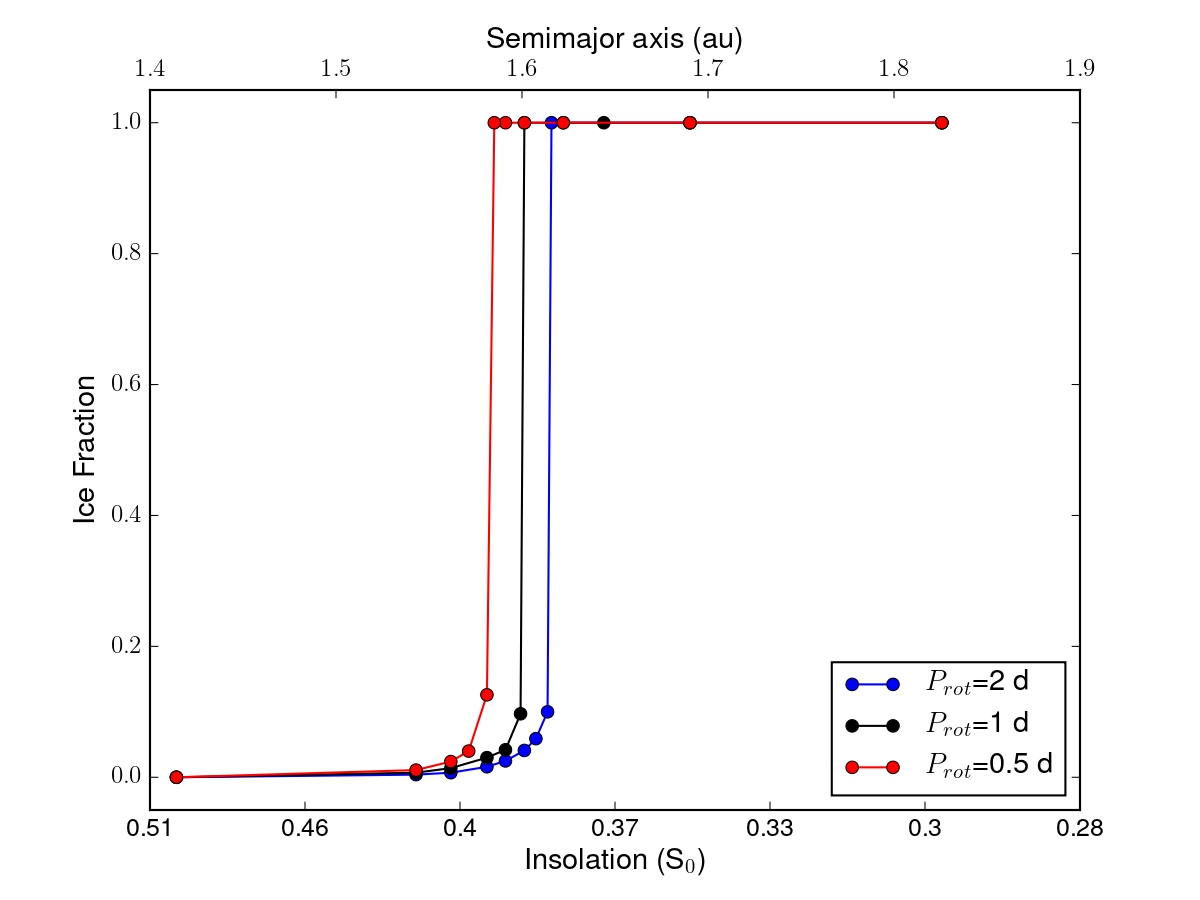
Fig. 3. Dependence on planetary rotation period rotation period, Prot, of the fractional ice coverage calculated at the outer edge of the HZ. The results were obtained for an Earth-like planet with a CO2-dominated, maximum greenhouse atmosphere, the remaining parameters being fixed to Earth values.
REFERENCES
Grimm S. L., Heng K., 2015, HELIOS-K: Opacity Calculator for Radiative Transfer (ascl:1503.004)
Grimm S. L., et al., 2021, ApJS, 253, 30
Kasting J. F., Whitmire D. P., Reynolds R. T., 1993, Icarus, 101, 108
Leconte J., Forget F., Charnay B., Wordsworth R., Pottier A., 2013, Nature, 504, 268
Malik M., et al., 2017, AJ, 153, 56
Malik M., Kitzmann D., Mendonça J. M., Grimm S. L., Marleau G.-D., Linder E. F., Tsai S.-M., Heng K., 2019, AJ, 157, 170
Simonetti P., Vladilo G., Silva L., Maris M., Ivanovski S. L., Biasiotti L., Malik M., von Hardenberg J., 2022, ApJ, 925, 105
Vladilo G., Murante G., Silva L., Provenzale A., Ferri G., Ragazzini G., 2013, ApJ, 767, 65
Vladilo G., Silva L., Murante G., Filippi L., Provenzale A., 2015, ApJ, 804,50
Wolf E. T., Toon O. B., 2014, Geophys. Res. Lett., 41, 167
Wolf E. T., Toon O. B., 2015, Journal of Geophysical Research (Atmospheres), 120, 5775