Introduction: Although Mars is now a cold, dry planet, the geological record shows that, early in its history, Mars not only went through episodes of wet-dry cycling [1], in which Mars could maintain liquid water on its surface for sustained periods of time, but was also volcanically active [2]. As a high-pressure CO2 and H2O atmosphere alone could not induce the required annually-averaged temperatures for liquid water given the brightness of the Sun at the time [3,4], one hypothesis suggested sulphur dioxide (SO2) and hydrogen sulphide (H2S) emitted by active volcanoes on the surface of Mars as a source of greenhouse warming [5]. Later studies then suggested that any greenhouse warming from SO2and H2S would be negated by the cooling effect of sulphuric acid (H2SO4) and elemental polysulphur (S8) clouds that would result from the reaction of SO2and H2S with water vapour in the atmosphere. However, these studies either relied on photochemical models in 1-D [6,7], which neglect spatial variations in cloud formation, or simple parametrisations of sulphur which do not adequately account for the formation timescales of H2SO4and S8 clouds [8]. All of these factors result in major uncertainties in the magnitude and duration of any warming or cooling on early Mars.
We therefore wish to investigate, using a 3-D Global Climate Model (GCM), how cycles of emission, reaction, condensation and deposition of sulphur would have affected the radiative balance of Mars, and hence the timescales of any volcanically-induced warming and cooling cycles that took place on Mars. In particular, we wish to observe whether the finite timescales of formation of H2SO4 and S8 clouds were significant enough to allow for a short period of time just following a volcanic eruption in which SO2 and H2S greenhouse warming could dominate over atmospheric cooling from H2SO4 and S8 clouds.
Method: We present the first implementation of the sulphur cycle on early Mars in a 3-D Global Climate Model (the Generic Planetary Climate Model (PCM) [9]) that takes a number of processes, most notably atmospheric chemistry, into account as shown in Figure 1. We simulate volcanic emission of sulphur according to a point surface flux of SO2, H2S, S2, HCl, CO and H2 as per the thermodynamic constraints on silicate partitioning in the Martian mantle [2], with a more reducing mantle favouring emission of H2S and S2, and a more oxidising mantle favouring SO2. Assuming a background atmosphere of 95% carbon dioxide and variable water vapour [4], we then simulate atmospheric chemistry according to 270 reactions that take odd-hydrogen, sulphur, nitrogen and chlorine chemistry into account [6,10-12], with the end products being H2SO4 (favoured in an oxidising atmosphere) and S8 (favoured in a reducing atmosphere). These two molecules then condense out of the atmosphere and are deposited onto the ground.
Although H2SO4 and H2O are expected to condense out together, a complex microphysical model involving binary H2SO4-H2O condensation is difficult to implement due to the lack of knowledge of the density of cloud condensation nuclei in the early Martian atmosphere, as well as the lack of laboratory constraints on microphysical parameters at the low atmospheric temperatures predicted for early Mars. We therefore assume a constant cloud particle radius and ratio of H2SO4 to H2O, and model condensation according to diffusion-limited growth [13] in order to allow for supersaturation of H2SO4 in the atmosphere and thereby delay the onset of the anti-greenhouse effect of H2SO4 clouds as much as possible. 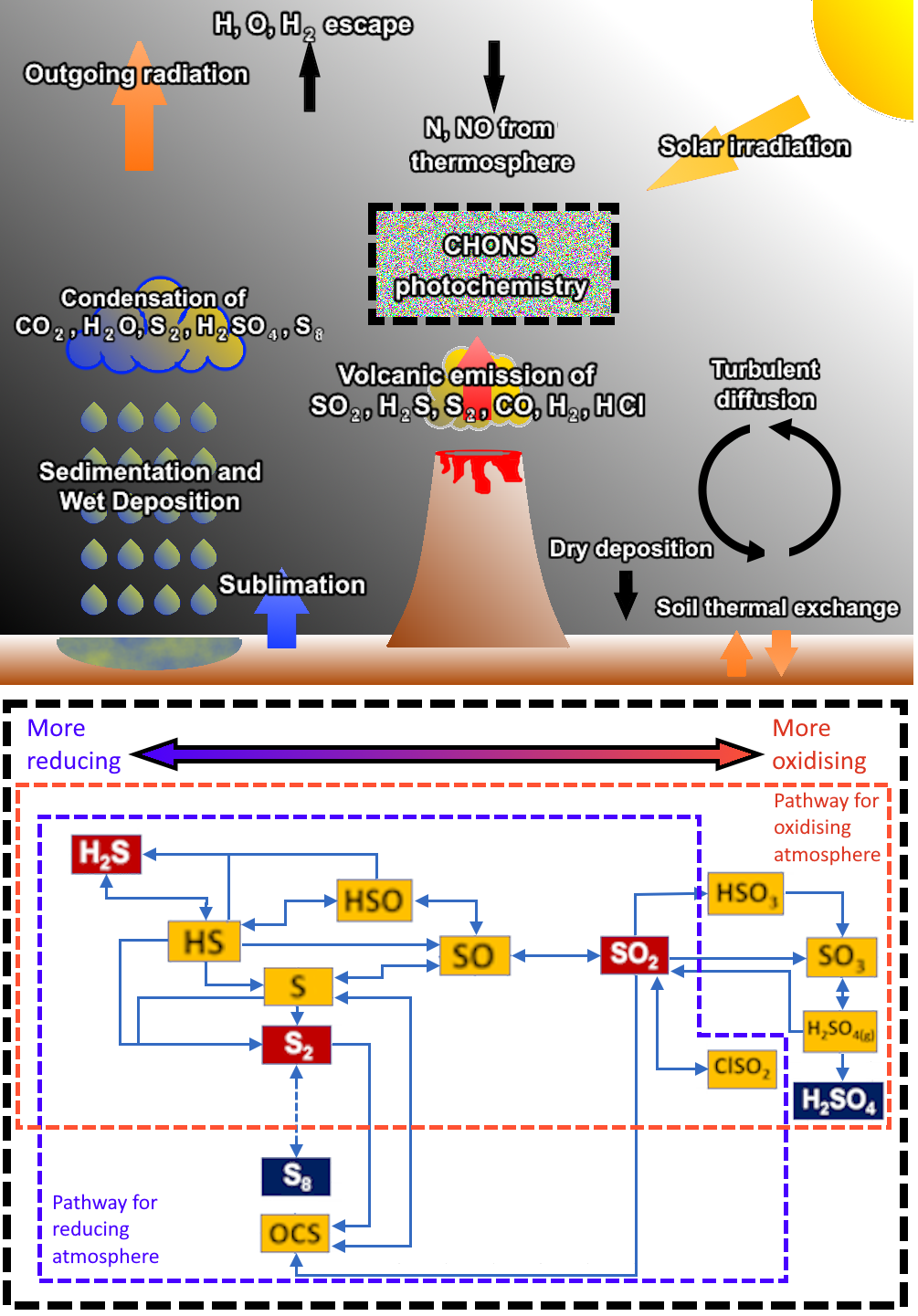
Figure 1. (top) a diagram of the major processes included in our model of the sulphur cycle, (bottom) the major photochemical pathways involved in the production of S8 and H2SO4 from outgassed SO2, H2S and S2 based on the redox state of the atmosphere.
Results: We confirm the results of [7,8] and find that the amount of greenhouse warming induced by volcanic SO2and H2S emission is both too weak and too short to melt liquid water on the surface of Mars. Although the anti-greenhouse effect from H2SO4 and S8 cloud formation can be delayed by increasing the atmospheric pressure, the increased thermal inertia of the atmosphere also delays the greenhouse effect from SO2 and H2S (Figure 2). A particularly large eruption can even induce runaway cooling of the atmosphere, eventually leading to atmospheric collapse as CO2 is no longer stable in the atmosphere in its gaseous form. We are unable to mitigate this either by changing the oxygen fugacity and water content of the Martian mantle, or the microphysical properties of the binary H2SO4-H2O condensate cloud particles.
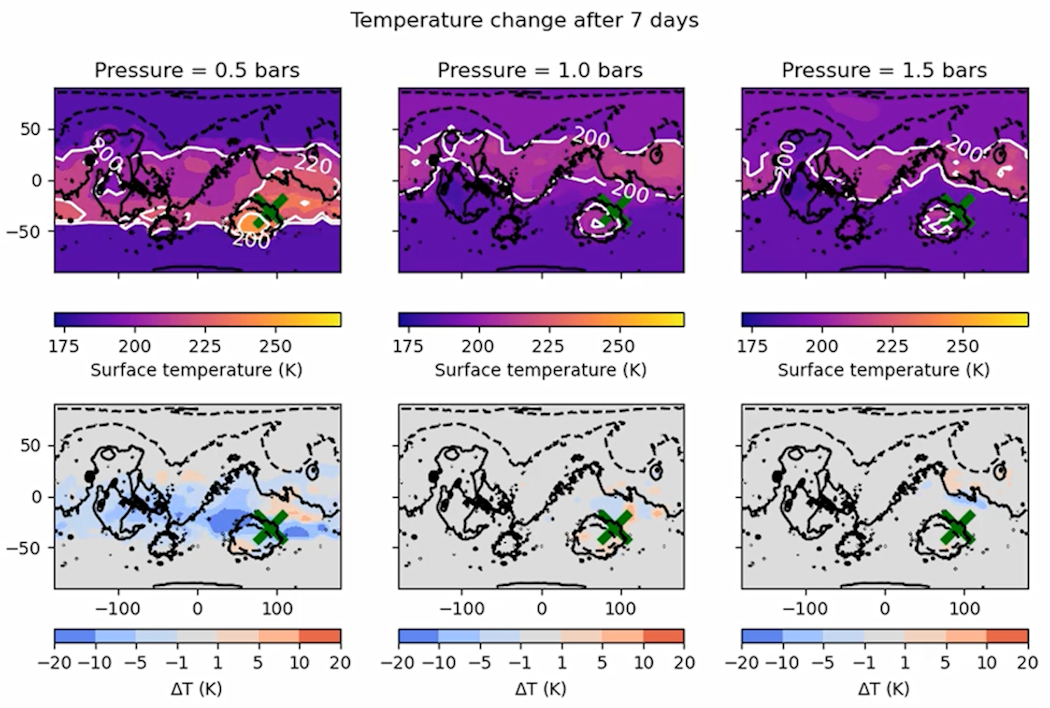
Figure 2. Simulation of a volcanic outgassing event (at the green cross) starting from three different average surface pressures, (top) surface temperature 7 days after the event and (bottom) increase in temperature relative to scenario where no eruption took place.
Acknowledgments: This work was carried out at the Jet Propulsion Laboratory California Institute of Technology under a contract with NASA. We recognize support for this project from NASA grant 20-SSW20-0086.
References: [1] Rapin, W. et al. (2023) Nature, 620, 299-302. [2] Gaillard, F. et al. (2013) Space Sci. Rev., 174, 251-300. [3] Forget, F. et al. (2013) Icarus, 222, 81-99. [4] Wordsworth, R. et al. (2015) J. Geophys. Res. Plan., 120, 1201-1219. [5] Yung, Y. L. et al. (1997) Icarus, 130, 222-224. [6] Johnson, S. S. et al. (2009) J. Geophys. Res. Plan., 114, E11011. [7] Tian, F. et al. (2010) EPSL, 295, 412-418. [8] Kerber, L. et al. (2015) Icarus, 261, 133-148 [9] Forget, F. et al. (1999) J. Geophys. Res., 104, 24155-24176. [10] Catling, D. C. et al. (2010) J. Geophys. Res., 115, E00E11. [11] Sholes, S. F. et al. (2017). [12] Stolzenbach, A. et al. (2023) Icarus, 395, 115447. [13] Hu, R. et al. (2012) ApJ, 761, 166.