Introduction: The search for organic compounds of biological origin in extraterrestrial environments is one of the major objectives of space missions. However, the interpretations of the origins (biological or otherwise) of organic matter in extraterrestrial environments is a highly complex process. The same concern is encountered when probing for the oldest traces of life on Earth. The search for by-products of potential biological activity such as bio-minerals can be an interesting alternative to decipher biological from non-biological organic compounds. Many minerals of biological origin have been identified on present-day Earth, trapping organic compounds of biological origin, used as template for mineralisation [1], [2]. Therefore, it can be assumed that traces of biological activity could have been preserved more efficiently in the form of inorganic phases such as bio-minerals and potentially able to protect and preserve in their crystallographic structure, biological organic compounds.
Nevertheless, numerous studies also demonstrate the possibility of forming abiotic minerals by organo-mineralization (precipitated solely in the presence of organic molecules without any living organisms) [3], [4], [5]. On Earth, carbonate minerals have been widely produced by bio-, organo-mineralization as well as by inorganic mineralization processes throughout geological history. However, both bio- and organo-mineralization processes use organic compounds as nucleation sites for carbonation leading in both cases to organic-rich carbonates with occasionally similar characteristics [6]. Few studies have shown that the nature of organic compounds, including their physico-chemical properties but also their structural form (chirality) lead to the formation of organo-carbonates with various characteristics (e.g., mineralogies, morphologies) [4], [5], [7]. The presence of one or more COO- functional groups on molecules, which can form a weak bond with Ca2+ ions and thus induce carbonation, could have an influence on the properties of organo-carbonates produced.
The aim here is to investigate the formation of organo-carbonates in various environmental conditions and with various chemical compounds to assess the physico-chemical properties of a wide diversity of organo-carbonates. This could give us an insight into the carbonation mechanisms linked to the intrinsic properties of organic compounds, and enable us to study the impact of these organic compounds on the properties of the organo-carbonates. Data acquired on the organo-carbonates produced in this study are also compared with a dataset acquired on numerous abiotic carbonates and bio-carbonates, both natural and laboratory-synthesized [6], [8], with the aim of identifying relevant criteria for distinguishing carbonates according to their mineralization process and identify possible false positives biosignatures.
Methods: Organo-carbonate precipitation was carried out using the ammonium diffusion method, where carbon dioxide and ammonium, derived from the spontaneous decomposition of ammonium carbonate, are gradually dissolved in an aqueous solution containing CaCl2 and amino acids. Experiments took place at room temperature for 20 days, in desiccators filled with silica gel, ammonium carbonate and petri dishes each containing 30 ml of aqueous solution. The organo-carbonates obtained were studied using a wide range of analytical techniques to investigate their physico-chemical properties. Morphologies were assessed by scanning electron microscopy, mineralogies and structural properties by X-ray diffraction and infrared spectroscopy, and thermal stability and organic content by differential thermal analysis and Rock-eval analysis.
Results: Experiments carried out with amino acids (various concentrations, with enantiomeric forms, inert or oxygenated atmospheres) produced organo-carbonates with different physico-chemical properties, such as different mineralogies (i.e., various proportions of carbonate polymorphs: calcite, aragonite, vaterite), morphologies (i.e., rhombohedral, spherical, needle-shaped with smooth or grooved surfaces), organic matter contents and thermal stabilities. These data were compared with results obtained on abiotic and bio-carbonates (~30 samples) acquired with the same instruments and the same analytical methods [6], [8]. Preliminary results obtained from the analysis of 3 organo-carbonates (precipitated with L-glutamine, L-glutamic acid, L-aspartic acid) show a wide diversity of physico-chemical properties. Each organo-carbonate samples have various proportions of carbonate polymorphs depending on amino acids, different morphologies (Fig. 1 - A, B, C) and a thermal decomposition at high temperatures (~815-820°C) closer to abiotic carbonates than to natural and laboratory-synthesized bio-carbonates (Fig. 1 - D).
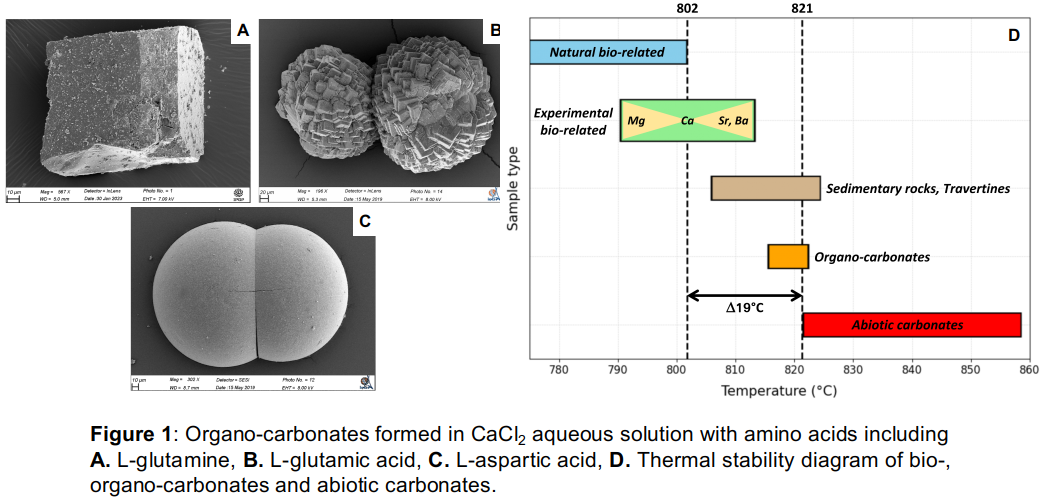
Discussion: Based on the preliminary results acquired on few organo-carbonates, minerals can have various morphologies and surface textures occasionally similar to bio-carbonates (spherical or needle-shaped) or abiotic carbonates (rhombohedral). It is therefore undeniable that morphology cannot be the only criterion for distinguishing carbonates according to their mineralization process. However, the spherical shape of some organo-carbonates could be a characteristic linked to the nature of the organic compound used as an organic template for mineralization. According to the physico-chemical properties of organic compounds more or less weak bonds between the Ca2+ and, for example, the COO- groups of certain amino acids might have been formed. Whereas, on the contrary, rhombohedral shapes may imply a limited use of certain organic compounds as triggers for the carbonation. To conclude on this hypothesis, many organo-carbonates will have to be synthesized in the presence of various amino acids, but also with other organic compounds having different physico-chemical properties (e.g., sugars, alkanes). Comparison between the properties of organo-carbonates and carbonates produced by bio- and inorganic mineralization (e.g., thermal stability, Fig.1-D) could be used as a criterion to distinguish bio-carbonates from organo-carbonates. However, to be able to assert the origin of a mineral, it is necessary to identify other criteria to corroborate these observations and thus accurately distinguish these two processes.
Acknowledgements: We gratefully acknowledge the technical support of S. BORENSZTAJN for the scanning electron microscopy (UPC, PARI platform - IPGP), S. NOWAK for X-ray diffraction analysis (UPC, X-ray platform) and A. CHEVILLOT-BIRAUD for the differential thermal analysis (UPC, ITODYS).
References:
[1] Tourney, J., & Ngwenya, B. T. (2009). Chemical Geology, 262(3-4), 138-146.
[2] Hoffmann, T. D., et al., (2021). Microbiology, 167(4), 001049.
[3] McMahon, S., & Cosmidis, J. (2022). Journal of the Geological Society, 179(2), jgs2021-050.
[4] Braissant, O., et al., (2003). Journal of Sedimentary Research, 73(3), 485-490.
[5] Xie, A. J., et al., (2005). Journal of Crystal Growth, 285(3), 436-443.
[6] Perron, A. , Paris, 2023.
[7] Wolf, S. E., et al., (2007). Angewandte Chemie International Edition, 46(29), 5618-5623.
[8] Perron, A., et al., (2023). Astrobiology, 23(4), 359-371.