ABSTRACT
The Global Dust Storm of Mars Year 34 was observed by the Mars Science Laboratory (MSL) in Gale crater, and many atmospheric effects were seen during its duration. Using atmospheric observations taken by MSL, water-ice cloud opacity and scattering phase function are calculated, allowing comparison of these quantities before and after the dust storm. The average phase function was calculated for the Mars Year 34 Aphelion Cloud Belt (ACB) season, before the global dust storm, and will be calculated for the ACB season following. Opacity calculations are computed throughout the Martian year. The results of these calculations will indicate any impacts the global dust storm may have on Martian water-ice clouds.
1. INTRODUCTION
The Martian atmosphere has long been the subject of study, both in situ and remotely, and as a result we are able to observe its dynamic nature. Water-ice clouds are one such dynamic feature, and their similarities to Earth-based clouds provide an opportunity to learn more not just about Martian weather but our own climate processes.
The Mars Science Laboratory (MSL) in Gale Crater has been observing cloud activity in the region for the entirety of its mission to date. Cloud movies captured by MSL can be analysed in order to determine optical parameters such as opacity[1] and scattering phase function[2]. The most important time of year for cloud activity around Gale Crater is the Aphelion Cloud Belt season, which tends to peak between solar longitudes (Ls) 60-100° and is characterised by an increase in equatorial cloud formation[3].
MSL has been in Gale Crater for nearly four Martian Years, and as a result has been able to observe the time-varying nature of the Martian climate on daily, seasonal, and annual scales. The presence of a global dust storm in 2018 (Mars Year 34) gave further opportunity to study not only the event itself, but its lasting effect on the Martian atmosphere.
2. BACKGROUND
2.1 The Mars Year 34 Global Dust Storm
The global dust storm of Mars Year (MY) 34 was a planet encircling dust storm which lasted from about Ls~188° to Ls~250°[4]. Global dust storms typically occur every 3-4 years[5] and significantly impact the atmosphere, causing temperature and pressure fluctuations[4] and an increase in water vapour in the middle atmosphere[6]. While the decrease in visibility made it difficult to observe clouds during the global dust storm, a before-and-after comparison of cloud opacity and scattering phase function provide understanding about longstanding atmospheric effects of global dust storms.
2.2 MSL Atmospheric Movies
The navigation cameras onboard MSL take two types of movies year-round, Zenith Movies (ZM) and Suprahorizon Movies (SHM), and a third observation during the ACB season, the Phase Function Sky Survey (PFSS). ZM and SHM are both 8 frame movies taken over 6 minutes, with ZMs pointing to the Zenith and SHMs pointing just above the crater rim. The PFSS is a mosaic of 9 3-frame movies at a variety of pointings making a dome surrounding the rover. In order to better identify clouds, all three types of movies undergo mean frame subtraction, a process by which an average frame is subtracted from each frame of the movie, leaving only the time variable component. This can be seen in Figure 1.
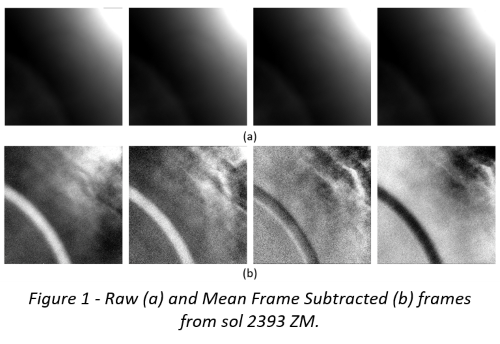
3. METHODS
ZM and SHM can be analysed to determine cloud optical thickness, which has been computed for the first two Martian Years of MSL’s mission (Ls=160° of MY 31 to Ls=160° of MY 33)[7]. After mean frame subtraction, a radiance map (Figure 2) is made of a single frame of the movie, which is examined manually for a region containing cloud and empty sky, giving a variation in spectral radiance (Iλ,VAR) which is then used to compute the optical thickness. During the ACB season, the high-cloud formula, derived by Kloos et al, is used to estimate opacity, which assumes clouds are high, optically thin, and composed of water-ice crystals[1].
The PFSS was first instituted in MY 34, and an average scattering phase function determined for that year[2]. As with optical thickness, Iλ,VAR is determined from a radiance map for each movie and used to compute the phase function, using the formula derived by Cooper et al[2]. With the MY 35 ACB season ending in February of 2020, there is now another complete set of 26 PFSS observations, giving 234 total movies.
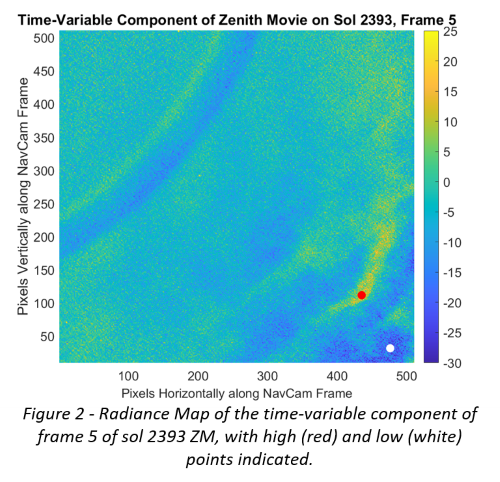
4. RESULTS AND DISCUSSION
Currently ZM and SHM have been processed and have radiance maps from sol 1706 to sol 2748, a total of 466 movies. All of these must be checked manually for quality, and to identify high and low radiance points. This process is ongoing. Following the calculation of optical thickness for all cloud movies, the catalogue of MSL cloud opacities will be extended from two Martian years to four.
In addition, the 234 PFSS movies must likewise be checked, a process that is nearing completion at time of writing. By the time of the conference, it is anticipated that an average phase function for MY 35 will be calculated, allowing comparison with that of MY 34. Due to the larger size of the opacity dataset, opacity calculations are anticipated to take more time.
These observations will allow us to better understand not only the lasting atmospheric effects of global dust storms, but also will extend our understanding of variability in water-ice cloud optical properties over larger timescales.
REFERENCES
[1] Kloos, J.L, et al, Adv. Space Res., 2016.
[2] Cooper, B.A., et al, P&SS, 2019.
[3] Wolff, M.J., et al, JGR, 1999.
[4] Guzewich, S.D. et al, Geophys. Res. Lett., 2019.
[5] Zurek, R.W., and Martin, L.J., JGR, 1993.
[6] Aoki, S.A., et al, JGR-Planets, 2019.
[7] Kloos, J.L. et al, JGR-Planets, 2018.