Phosphorous, P, is a key biological element with major roles in replication, information transfer, and metabolism (Maciá, 2005). Interplanetary dust particles contain ∼0.1% P by weight, and meteoric ablation in the 1 μbar region of a planetary upper atmosphere will inject P, PO etc. into the atmosphere. These species will then undergo atmospheric processing before deposition at the surface. Orthophosphate (oxidation state +5) is the dominant form of inorganic P at the Earth’s surface; however, due to the low water solubility and reactivity of P(+5) salts, they have a poor bio-availability (Redfield, 1958). In contrast, less oxidised forms of P (particularly oxidation state +3) are far more bio-available. It has been suggested that these reduced forms of P may have originated from extra-terrestrial material that fell to Earth during the heavy bombardment period.
Previous studies have focused on the direct delivery of P to the surface in meteorites, to undergo processing through aqueous phase chemistry (Pasek, 2008). In contrast, the atmospheric chemistry of P has so far been ignored. Most of the mass of extra-terrestrial P entering a planetary atmosphere is carried by interplanetary dust particles (IPDs) with a mass of ~5 μg and a radius of ~100 μm (Carrillo-Sánchez et al., 2016). A substantial fraction of these particles ablate due to aerobraking, at heights of ~80 km on Mars, 92 km on Earth, and 115 km on Venus (Carrillo-Sánchez et al., 2020). The vaporized P atoms will then undergo chemical processing to form a variety of compounds, in which P may exist in different oxidation states due to the presence of both oxidizing and reducing agents in the upper atmospheres of the terrestrial planets. Here we present the first study of the meteoric ablation of phosphorous, and its subsequent chemical processing to form a variety of compounds including P in the biologically important P(+3) state.
The ablation of PO – relative to Ca – was studied in the Leeds Meteoric Ablation Simulator, and a new version of our Chemical Ablation Model (CABMOD) was developed to include the thermodynamics of P oxides in a molten meteoroid (Carrillo-Sánchez et al., 2020). The speciation of P in anhydrous chondritic porous Interplanetary Dust Particles was made by K-edge X-ray absorption near edge structure (XANES) spectroscopy at the Diamond Synchrotron; this work demonstrated that P mainly occurs in phosphate-like domains. An astronomical dust model which predicts the amounts of dust in the inner solar system produced by Jupiter Family comets, the asteroid belt, and Long Period comets, was then combined with CABMOD to predict the injection rate profile of P, PO and PO2 into the atmospheres of Mars, Earth and Venus - the Meteoric Input Function (Carrillo-Sánchez et al., 2020).
We then measured the kinetics of P, PO and OPO with a variety of atmospheric constituents using the laser flash photolysis/laser induced fluorescence technique (Douglas et al., 2019; Douglas et al., 2020). Electronic structure (ab initio quantum) calculations were also combined with statistical rate theory to produce an atmospheric chemistry network for phosphorus (Figure 1). This network shows the pathways from OPO to H3PO3 and H3PO4. These phosphorus-oxy acids then combine with the metal-containing molecules (e.g. NaHCO3, FeOH), also produced by meteoric ablation, to form metal phosphites and phosphates. These compounds polymerize into nm-sized meteoric smoke particles, which are transported down to the surface over several years (Dhomse et al., 2013).
This chemistry, together with the phosphorus MIF, was then put into the global chemistry-climate model WACCM. Figure 2 shows the predicted percentage of P(+3) to total P in meteoric smoke particles above 60 km, as a function of latitude and season. This study demonstrates that biologically important phosphorous acid (H3PO3) can form and then react with meteoric metal species to generate bio-available metal hydrogen phosphites. The global input of reduced P(+3) to the Earth’s surface is estimated to be around 600 kg year-1.
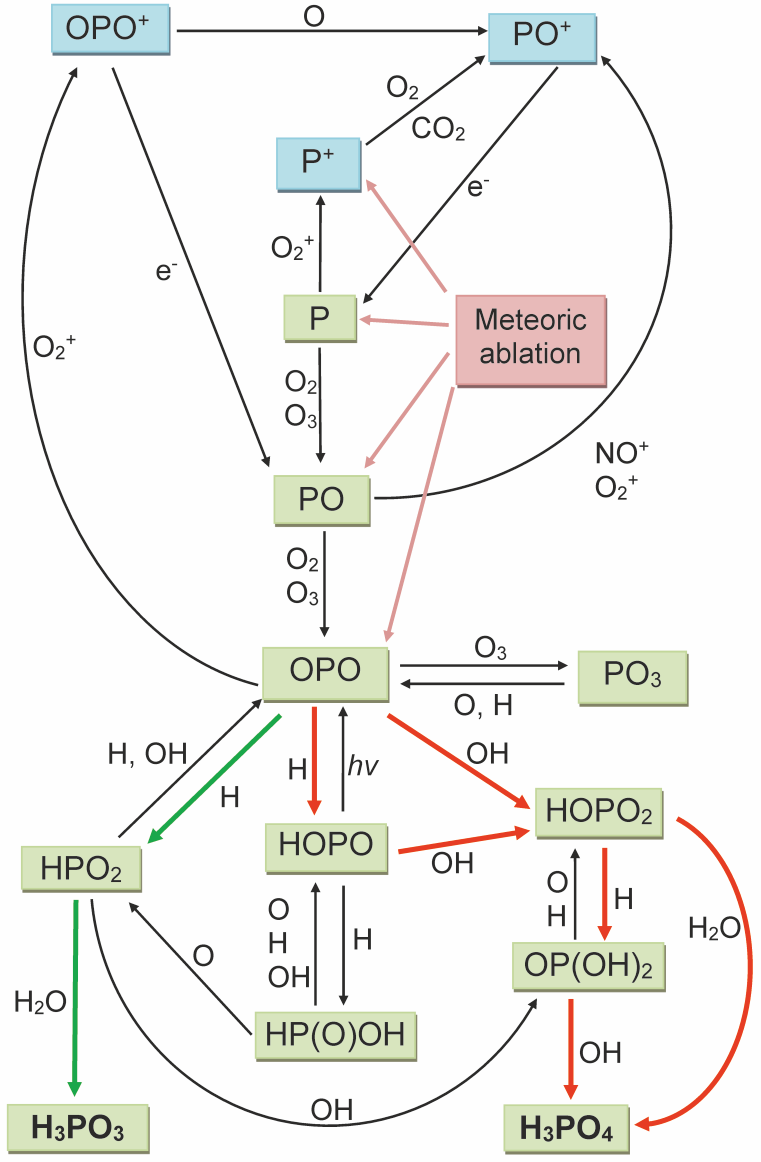
Figure 1. Schematic diagram of the chemistry of phosphorus in the earth’s mesosphere and lower thermosphere. The green and red arrows indicate the important pathways from OPO to H3PO3 and H3PO4, respectively.
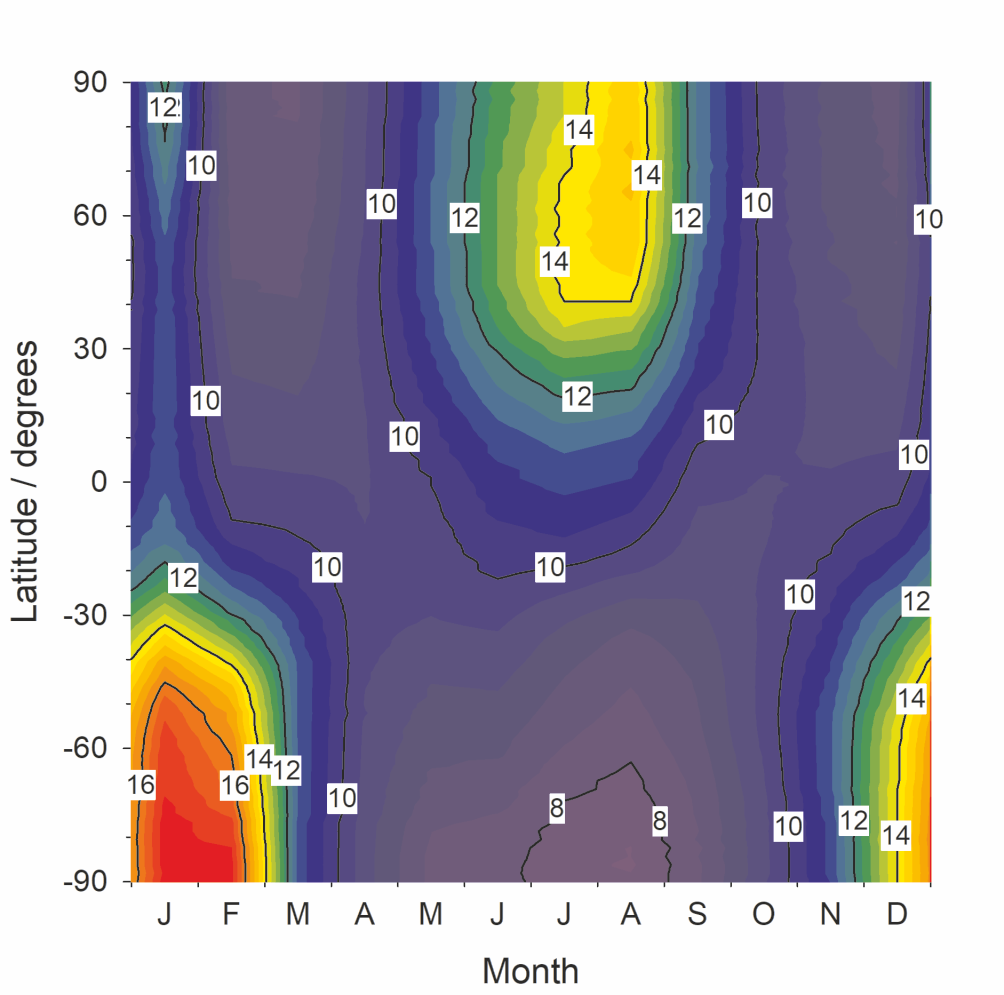
Figure 2. Percentage of P(+3) to total P in meteoric smoke particles above 60 km, as a function of latitude and month (averaged over 3 years).
References
Carrillo-Sánchez, J.D., Bones, D.L., Douglas, K.M., Flynn, G.J., Wirick, S., Fegley, B., Araki, T., Kaulich, B., Plane, J.M.C., 2020. Injection of meteoric phosphorus into planetary atmospheres. Planet. Space Sci. 187, art. no. 104926.
Carrillo-Sánchez, J.D., Nesvorny, D., Pokorny, P., Janches, D., Plane, J.M.C., 2016. Sources of cosmic dust in the Earth's atmosphere. Geophys. Res. Lett. 43, 11979-11986.
Dhomse, S.S., Saunders, R.W., Tian, W., Chipperfield, M.P., Plane, J.M.C., 2013. Plutonium-238 observations as a test of modeled transport and surface deposition of meteoric smoke particles. Geophys. Res. Lett. 40, 4454-4458.
Douglas, K.M., Blitz, M.A., Mangan, T.P., Plane, J.M.C., 2019. Experimental Study of the Removal of Ground- and Excited-State Phosphorus Atoms by Atmospherically Relevant Species. J. Phys. Chem. A 123, 9469-9478.
Douglas, K.M., Blitz, M.A., Mangan, T.P., Western, C.M., Plane, J.M.C., 2020. Kinetic Study of the Reactions PO + O2 and PO2 + O3 and Spectroscopy of the PO Radical. J. Phys. Chem. A 124, 7911-7926.
Maciá, E., 2005. The role of phosphorus in chemical evolution. Chemical Society Reviews 34, 691-701.
Pasek, M.A., 2008. Rethinking early Earth phosphorus geochemistry. Proceedings of the National Academy of Sciences 105, 853-858.
Redfield, A.C., 1958. The biological control of chemical factors in the environment. American Scientist 46, 205-221.