Isotope ratios manifest escape of the atmosphere to space and thus evolution of the climate through time, because lighter isotopes are removed from the atmosphere at a faster rate than heavier isotopes. Therefore, atmospheres experiencing extensive loss are enriched in the heavier isotopes of escaping elements. This occurs because the upper atmosphere, the reservoir of gas which escapes to space, is enriched in lighter isotopes due to diffusive separation and lighter isotopes have smaller escape energies. The Mars Atmosphere and Volatile EvolutioN (MAVEN) Neutral Gas and Ion Mass Spectrometer (NGIMS) measures the abundances of a number of molecular isotopologues, enabling an investigation of isotope ratios in the upper atmosphere of Mars.[1,2]
Using NGIMS data, we investigate both the magnitude and variation of isotope ratios in the upper atmosphere of Mars. The isotope ratios measured in this region of the atmosphere vary due to a number of processes in addition to atmospheric escape, such as diffusive separation, atmospheric transport, condensation, and photochemistry. MAVEN NGIMS has now collected thousands of vertical profiles of major isotope ratios, such as those of oxygen and carbon, over a wide range of atmospheric conditions: over the entire Martian day, across most latitudes, and through the seasons of multiple Mars years.
An isotope ratio is obtained from the NGIMS data by calculating the ratio of detector count rates in two mass per charge (m/z) channels. Then, a δ value is calculated relative to typical references standards such as Vienna Pee Dee Belemnite or Vienna Standard Mean Ocean Water. Mean profiles of the isotope ratios are produced from sequential orbits to improve the signal-to-noise ratio. Individual orbit profiles are also binned on local time, latitude, and season, to produce maps of the variation of each of the ratios. Uncertainties in these measurements must be carefully calculated to validate the accuracy of the relatively small differences measured between the isotope ratios of Martian atmospheric species and the terrestrial reference standards.
The isotope ratios of C and O in CO2 are of interest because CO2 is the main atmospheric species and thus the predominant atmospheric reservoir of C and O atoms which may escape to space. In the upper atmosphere, above the homopause, the ratios of heavier isotopes to lighter isotopes decrease with height due to diffusive separation, because lighter species have larger scale heights than heavier species. The expected decrease with height of measured isotope ratios is observed, for example, in the δ13C ratio in CO2 measured by NGIMS using m/z channels 45, corresponding to 13C16O2, and 44 corresponding to 12C16O2 (Figure 1). In addition, significant variation of δ13C in CO2 is found with Martian local time (Figure 2). This trend follows the trend in temperature.[3] Where the atmosphere is cooler, δ13C is smaller, and where the atmosphere is warmer δ13C is larger. The observed trend can be explained by a process which begins with heating on the dayside, causing the upward transport from below of gas relatively enriched in the heavier isotope, followed by subsolar-to-antisolar flow and downwelling on the nightside, causing the downward transport from above of gas relatively enriched in the lighter isotope on the cooler nightside.

Figure 1. A mean vertical profile of δ13C measured in CO2 relative to Vienna Pee Dee Belemnite (VPDB).
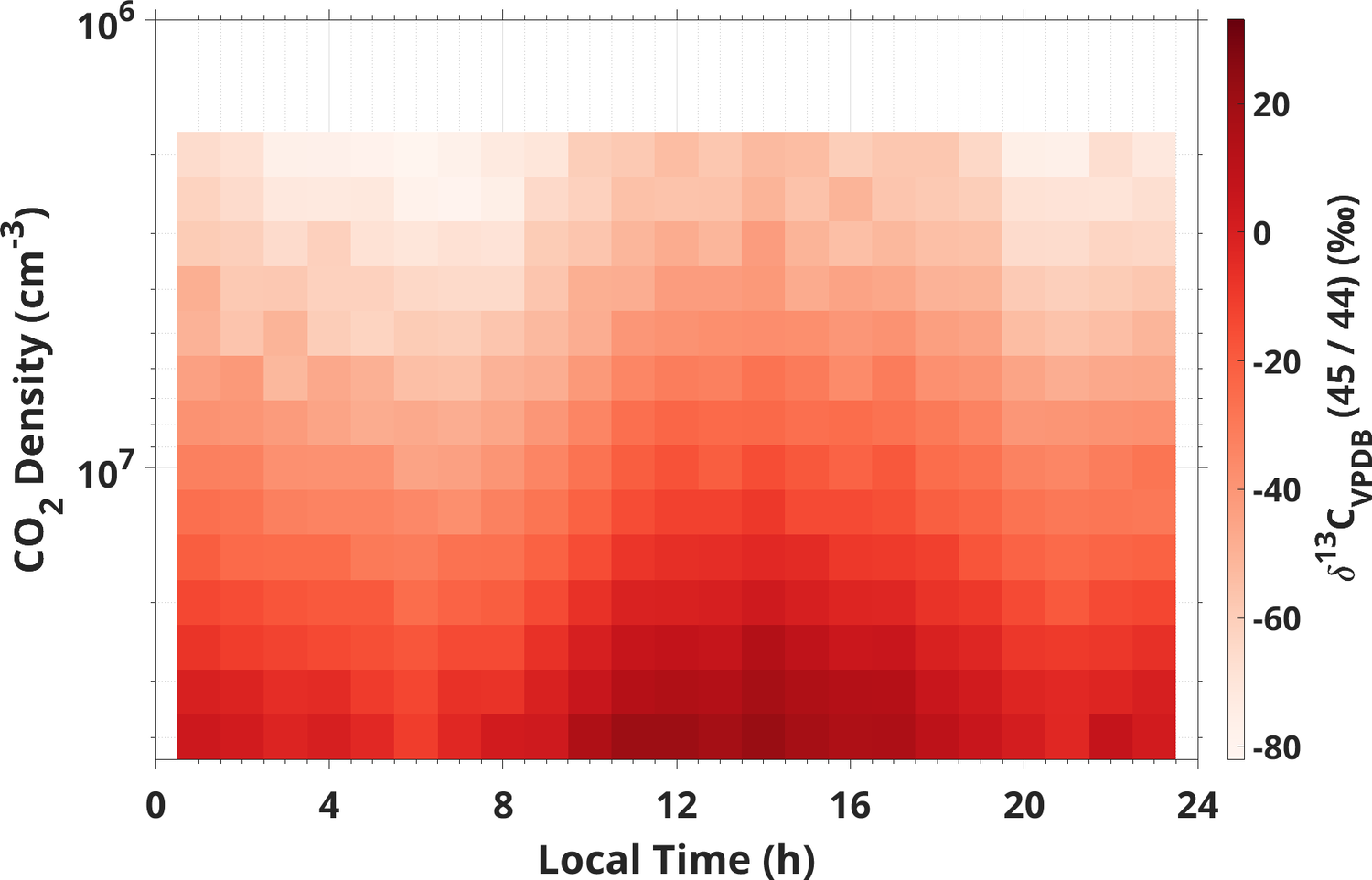
Figure 2. The local time variation of δ13C in CO2 relative to Vienna Pee Dee Belemnite (VPDB).
Comparisons can be made between the MAVEN NGIMS measurements of the isotope ratios in the upper atmosphere and measurements of these isotope ratios in the lower, middle, and upper atmosphere collected by Sample Analysis at Mars (SAM) on the NASA Curiosity rover,[4-5] Nadir and Occulation for MArs Discovery (NOMAD) and Atmospheric Chemistry Suite (ACS) onboard the ESA Trace Gas Orbiter,[8] and the NASA Viking Upper Atmospheric Mass Spectrometers.[9] These comparisons provide opportunities for the validation of NGIMS observations and provide further insight into the processes affecting the magnitude and variation of these isotope ratios from the surface to the top of the atmosphere.
References
[1] Jakosky, B. M., Lin, R. P., Grebowsky, J. M., Luhmann, J. G., Mitchell, D. F., Beutelschies, G., et al. (2015). The Mars Atmosphere and Volatile Evolution (MAVEN) Mission. Space Science Reviews, 195(1), 3–48. doi:10.1007/s11214-015-0139-x
[2] Mahaffy, P. R., Benna, M., King, T., Harpold, D. N., Arvey, R., Barciniak, M., et al. (2015). The Neutral Gas and Ion Mass Spectrometer on the Mars Atmosphere and Volatile Evolution Mission. Space Science Reviews, 195(1–4), 49–73. doi:10.1007/s11214-014-0091-1
[3] Stone, S. W., Yelle, R. V., Benna, M., Elrod, M. K., & Mahaffy, P. R. (2018). Thermal Structure of the Martian Upper Atmosphere From MAVEN NGIMS. Journal of Geophysical Research: Planets, 123(11), 2842–2867. doi:10.1029/2018je005559
[4] Mahaffy, P. R., Webster, C. R., Atreya, S. K., Franz, H. B., Wong, M. H., Conrad, P. G., et al. (2013). Abundance and Isotopic Composition of Gases in the Martian Atmosphere from the Curiosity Rover. Science, 341(6143), 263–266. doi:10.1126/science.1237966
[5] Webster, C. R., Mahaffy, P. R., Flesch, G. J., Niles, P. B., Jones, J. H., Leshin, L. A., et al. (2013). Isotope Ratios of H, C, and O in CO2 and H2O of the Martian Atmosphere. Science, 341(6143), 260–263. doi:10.1126/science.1237961
[6] Wong, M. H., Atreya, S. K., Mahaffy, P. R., Franz, H. B., Malespin, C., Trainer, M. G., et al. (2013). Isotopes of nitrogen on Mars: Atmospheric measurements by Curiosity’s mass spectrometer. Geophysical Research Letters, 40(23), 6033–6037. doi:10.1002/2013GL057840
[7] Atreya, S. K., Trainer, M. G., Franz, H. B., Wong, M. H., Manning, H. L. K., Malespin, C. A., et al. (2013). Primordial argon isotope fractionation in the atmosphere of Mars measured by the SAM instrument on Curiosity and implications for atmospheric loss. Geophysical Research Letters, 40(21), 5605–5609. doi:10.1002/2013GL057763
[8] Alday, J., Trokhimovskiy, A., Irwin, P. G. J., Wilson, C. F., Montmessin, F., Lefévre, F., et al. (2021). Isotopic fractionation of water and its photolytic products in the atmosphere of Mars. Nature Astronomy, 5(9), 943–950. doi:10.1038/s41550-021-01389-x
[9] Nier, A. O., McElroy, M. B., & Yung, Y. L. (1976). Isotopic composition of the martian atmosphere. Science, 194(4260), 68–70. doi:10.1126/science.194.4260.68