Abstract
As a potential pro-am complement to professional Jovian ammonia observations, continuum-divided 645 nm ammonia absorption observations were made using a small telescope. This paper presents highlights of observations during 2020 and 2021. If this low-cost technique can be promulgated among amateurs, then routine atmospheric monitoring of Jupiter would reach a new level of sophistication.
Introduction
New microwave and MIR observations, along with models, reveal much about Jupiter's ammonia cycle at depth. For example, the Juno MWR instrument permits the retrieval of the average ammonia abundance to a depth of 100 bar [1]. Additional recent work has used MIR observations to probe to depths of several bars [2-3]. Similarly, there have been efforts at global retrievals using hyperspectral imaging in the optical and NIR [4-5]. Complementing these efforts have been notable improvements in the understanding of the ammonia optical and NIR absorption bands [6]. Slit spectrometry data extend an already long record [7]. Finally, recent work has shown the efficacy of imaging Jovian upper tropospheric features in the 645 nm ammonia absorption band [8], which the current paper expands upon.
Observations
Thirty-nine usable observing sessions were carried out during 2020-2021 from the author’s observatory in Denver, Colorado. Fig. 1 shows the observations versus System I longitude. It also depicts Juno perijoves. During July and September 2020 observations overlap with the longitudes observed by Juno on PJ28 and PJ29. The best adjacent observations in 2021 occurred in October (PJ37). Also, near Juno perijove (PJ36), the System 1 longitude range of 140-180 degrees was observed multiple times. This allows for observing the evolution of features in the Equatorial Zone (EZ).
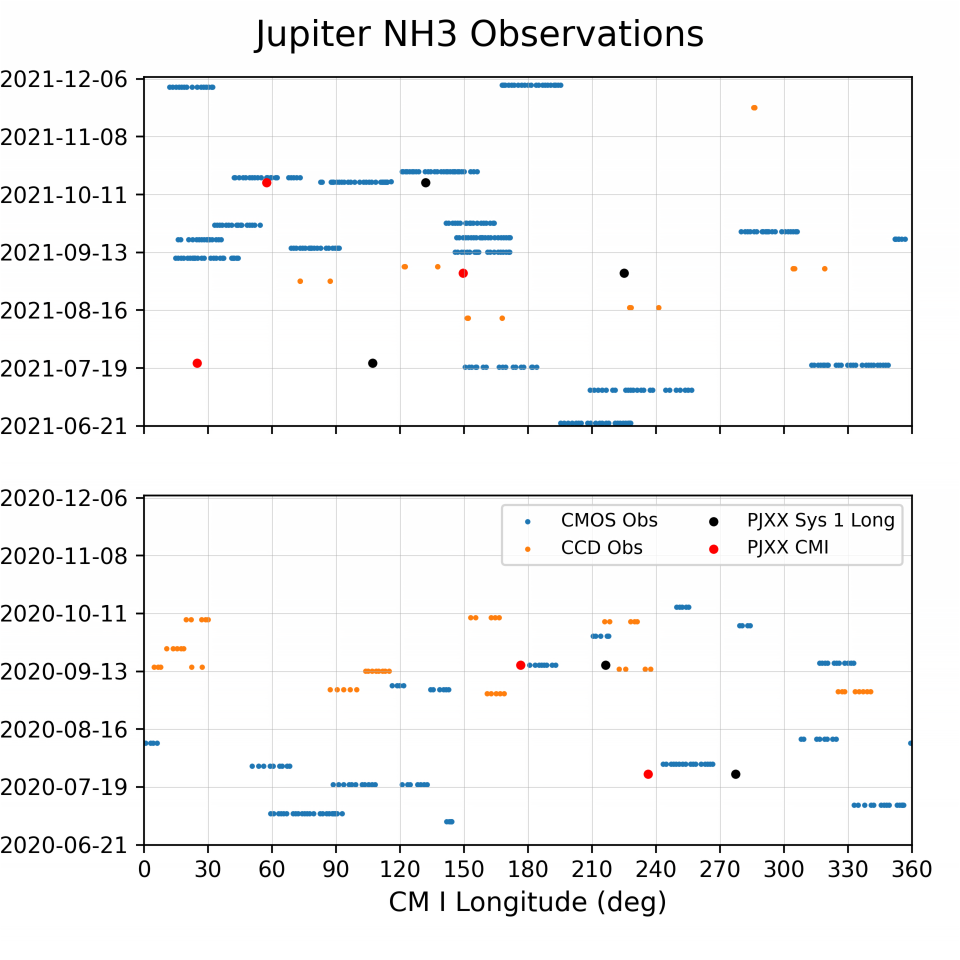
Figure 1: Observing sessions in 2020 (bottom) and 2021 (top). Individual images contributing to ammonia absorption observations are shown (CMOS: blue; CCD: orange). Juno perijove longitudes (Sys. 1) and Earth-facing central meridians (Sys. 1) at perijove are indicated in black and red respectively.
Highlights
Fig. 2 shows the NEB has reduced ammonia absorption, the EZ is enhanced, and the GRS is reduced. Note the correlation and lack of correlation with obvious visible features. There is a reduction correlated with the GRS, but the reduced absorption region from about 15-25N includes both bright and dark features.
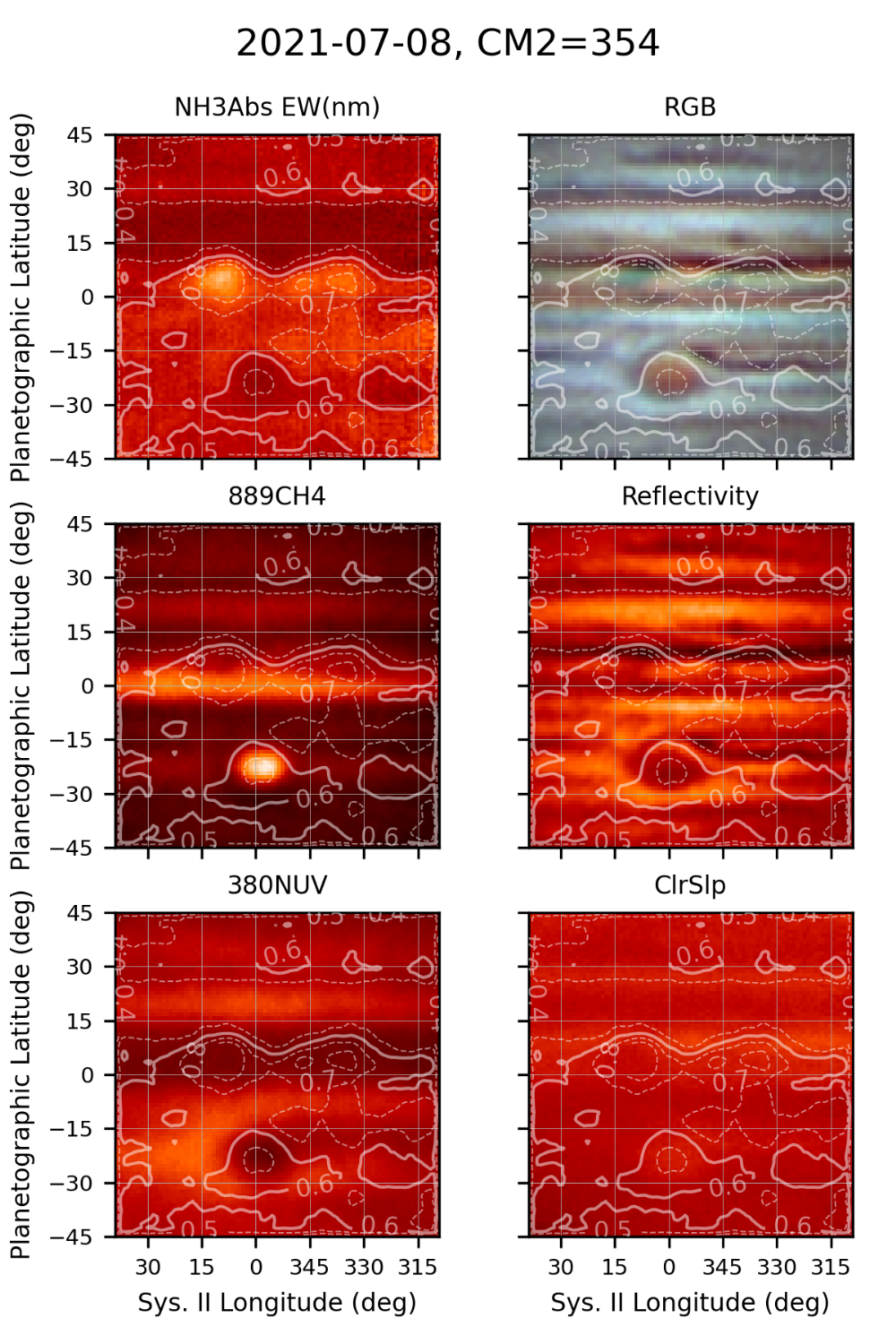
Figure 2: Ammonia absorption and context maps. Brightness scaling is arbitrary and adjusted for visual effect. Contour levels are estimated ammonia absorption equivalent width in nm. “ClrSlp” is relative color slope with redder areas shown as brighter.
Fig. 3 shows the EZ and NEB, including ammonia absorption enhancements near plumes and dark features. Dark features look deep into the atmosphere and the bright plumes represent high clouds.
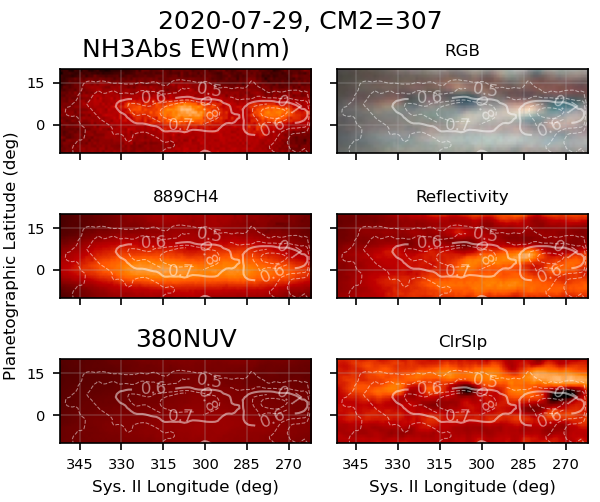
Figure 3: Same as Figure 4 but focused on the northern EZ.
The GRS is shown from July through September 2021 in Fig. 4. Ammonia absorption is reduced over the GRS due to the high-altitude scattering layer there. This reduction has also been noted in spectroscopic observations [7].
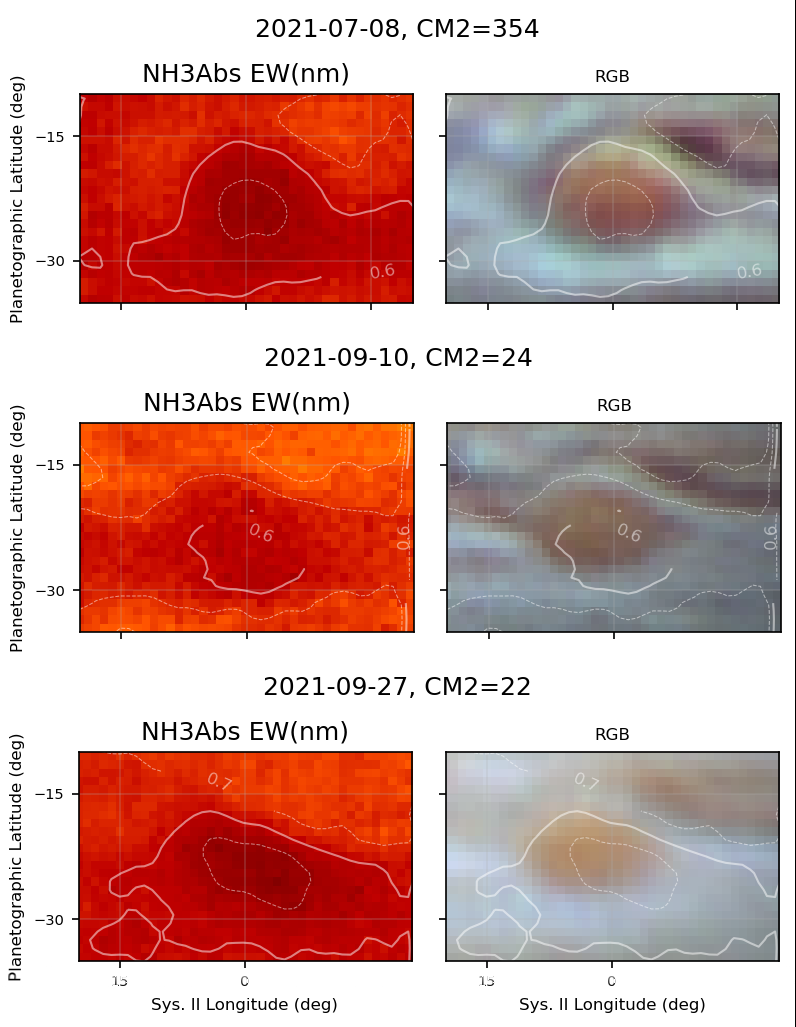
Figure 4: Reduced ammonia absorption over the Great Red Spot at three epochs in 2021.
Retrieval Potential
A scatter plot of 889 nm brightness versus 645 nm NH3 equivalent width (Fig. 5) shows the distributions of different latitude bands. High brightness in the methane band indicates higher cloud tops, which leads to a shorter absorption path. Thus, the GRS (high brightness red ‘tail’ in the 15-30S band) has a high reflecting layer. The ammonia EW is low, consistent with the short absorption path. The NEB (brown) shows uniformly low methane brightness, indicating deeper cloud tops, but also shows low ammonia absorption. This supports an actual depletion in ammonia abundance.
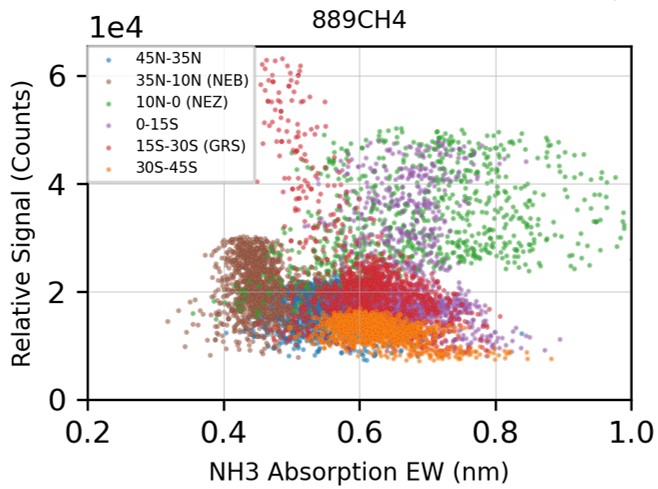
Figure 5: Scatter plot 889 nm methane relative signal versus 645 nm ammonia equivalent width for different meridional bands (2021-07-08, CM2 354 deg).
The scatter plot analysis suggests that a simple Reflecting Layer Model might provide meaningful first-order retrievals of atmospheric properties [9]. The goal during 2022 will be to retrieve reflectivity, cloud top pressure, and ammonia abundance by extending this model. In addition, observations will be shared on amateur collaboration websites.
Summary and Conclusion
This paper highlights two years of continuum-divided ammonia absorption imaging of Jupiter. The method shows detail inaccessible with other imaging techniques. The observations will be tested for utility in a simple atmospheric retrieval model and will be shared during the 2022 apparition.
References
[1] Guillot, T., et al. (2020), Storms and the Depletion of Ammonia in Jupiter: II. Explaining the Juno Observations, Journal of Geophysical Research (Planets), 125, e06404.
[2] Fletcher, L. N., et al. (2020), Jupiter's Equatorial Plumes and Hot Spots: Spectral Mapping from Gemini/TEXES and Juno/MWR, Journal of Geophysical Research (Planets), 125, e06399.
[3] Fletcher, L. N., et al. (2021), Jupiter's Temperate Belt/Zone Contrasts Revealed at Depth by Juno Microwave Observations, Journal of Geophysical Research (Planets), 126, e06858.
[4] Braude, A. S., P. G. J. Irwin, G. S. Orton, and L. N. Fletcher (2020), Colour and tropospheric cloud structure of Jupiter from MUSE/VLT: Retrieving a universal chromophore, Icarus, 338.
[5] Dahl, E. K., N. J. Chanover, G. S. Orton, K. H. Baines, J. A. Sinclair, D. G. Voelz, E. A. Wijerathna, P. D. Strycker, and P. G. J. Irwin (2021), Vertical Structure and Color of Jovian Latitudinal Cloud Bands during the Juno Era, The Planetary Science Journal, 2, 16.
[6] Irwin, P. G. J., N. Bowles, A. S. Braude, R. Garland, S. Calcutt, P. A. Coles, S. N. Yurchenko, and J. Tennyson (2019), Analysis of gaseous ammonia (NH3) absorption in the visible spectrum of Jupiter - Update, Icarus, 321, 572.
[7] Teifel', V. G., V. D. Vdovichenko, P. G. Lysenko, A. M. Karimov, G. A. Kirienko, N. N. Bondarenko, V. A. Filippov, G. A. Kharitonova, and A. P. Khozhenets (2018), Ammonia in Jupiter's Atmosphere: Spatial and Temporal Variations of the NH3 Absorption Bands at 645 and 787 Nm, Solar System Research, 52, 480.
[8] Hill, S. (2021), Experimental Observations of Jupiter in the Optical Ammonia Band at 645 nm, edited, pp. EPSC2021-2260.
[9] Mendikoa, I., S. Pérez-Hoyos, and A. Sánchez-Lavega (2012), Probing clouds in planets with a simple radiative transfer model: the Jupiter case, European Journal of Physics, 33, 1611.