Introduction: During its thirteen-year orbit around the Saturnian system (2004–2017), the Cassini mission produced an outstanding amount of data that made it possible to characterize Saturn, its rings, and its moons. Studying the composition and physical characteristics of Saturn's icy moons was one of the mission's main scientific objectives, aiming at understanding how different endogenic and exogenic processes interact to alter the moons' surfaces. The spectrophotometric properties of the MId-sized Saturnian icy Satellites (MISS: i.e., Mimas, Enceladus, Tethys, Dione, and Rhea) were extensively characterized in the 0.35–5.1 µm spectral range by the Visual and Infrared Mapping Spectrometer (VIMS, [1]) onboard the Cassini orbiter. This revealed surfaces dominated by water ice with variable amounts of contaminants, causing color changes (e.g., spectral reddening in the 0.35-0.55 µm interval), and albedo variations within and among the moons (Fig. 1).
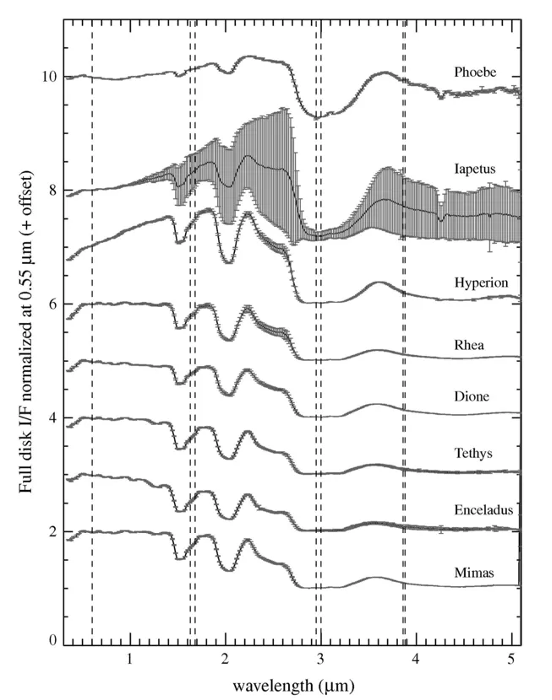
Figure 1. VIMS spectra of Saturn's icy moons, with the characteristic water ice bands at 1.5, 2., and 3 μm. The presence of non-icy compounds causes variations in albedo, VIS-NIR spectral slope, and UV downturn at 0.35-0.55 μm. Vertical dashed lines indicate VIMS' order sorting filter gaps. Adapted from [2].
By establishing appropriate spectral indicators (such as spectral slopes in the UV-VIS and VIS-NIR wavelength intervals and the depth of water ice absorptions) connected to regolith grain size and water ice/contaminant abundances, several studies examined the spectral and compositional variability of the MISS, providing qualitative trends of average properties [2, 3, 4] and at disk-resolved scales [5-7]. However, this approach was unable to fully characterize the compositional and physical properties from a quantitative standpoint, because this information is still at least partially entangled in the spectral indicators.
In an attempt to conduct a more quantitative analysis of the composition and physical characteristics of MISS's surfaces, VIMS hyperspectral data has been exploited through comparison with the outcomes of radiative transfer solutions (e.g., Hapke theory, 8), allowing to compute the spectra of different mixtures as a function of endmember abundances, mixing modalities, and grain size distributions. Such spectral modeling effort enables one to determine the spectral contribution of various compositional and physical properties to the observed spectral shape. In the case of MISS, similar attempts have been conducted in a small number of cases to constrain the composition of chosen terrains on selected targets (see [9] for Dione), or to determine the MISS compositional properties at full-disk scale [10, 2]. On the other hand, a systematic application of this methodology to perform a global compositional mapping from disk-resolved observations of MISS has not been performed yet.
In this paper, we set ourselves to this task, starting with the largest of Saturn's mid-sized icy moons, Rhea. To this end, we take advantage of VIMS photometrically-corrected disk-resolved data of the satellite, made available by [7]. This data, by minimizing the observation geometry bias, allows us to characterize the intrinsic specral and albedo variability of the surface and, by means of systematic spectral modeling, map the distribution of water ice and contaminants across the surface, along with the regolith grain size.
Method: we employ a VIMS photometrically-corrected spectral map of Rhea, adapting the methodology outlined in [7] (Figure 2, top panel). The spectral map is created by averaging photometrically corrected VIMS observations over a standard longitude-latitude grid with 0.5°x0.5° bin sampling. A hyperspectral cube with a spectrum assigned to each map position is the end product.
Using Hapke's theory, we conduct systematic spectral modeling for every bin in the spectral map. The surface is characterized as a mixture of water ice, a macromolecular organic compound (tholin, causing the UV absorption), and amorphous carbon (as a darkening contaminant), adopting a paradigm similar to previous modeling attempts [11].
Preliminary findings: we present a preliminary compositional map of Rhea in Figure 2 (middle panel). The spectral modeling results point to a surface composition dichotomy, where non-icy materials (carbon and tholin) are more abundant in the trailing hemisphere (longitudes 180°–360°) than in the leading one (longitudes 0°–180°), hosting larger amounts of water ice. The distribution of the various endmembers maps the albedo and color dichotomy of the surface, with a redder/darker trailing side contrasted to a bluer/brighter leading hemisphere (bottom panel of figure 2).
The inferred endmember spatial variability will be examined in light of the exogenous processes affecting Rhea's surface, such as photolysis, flux of charged particles driven by Saturn's magnetosphere, contamination of exogenic darkening material, and bombardment from E-ring grains [12], accounting for their longitudinal dependence, along with cratering and endogenous processes that shaped the surface of Rhea.
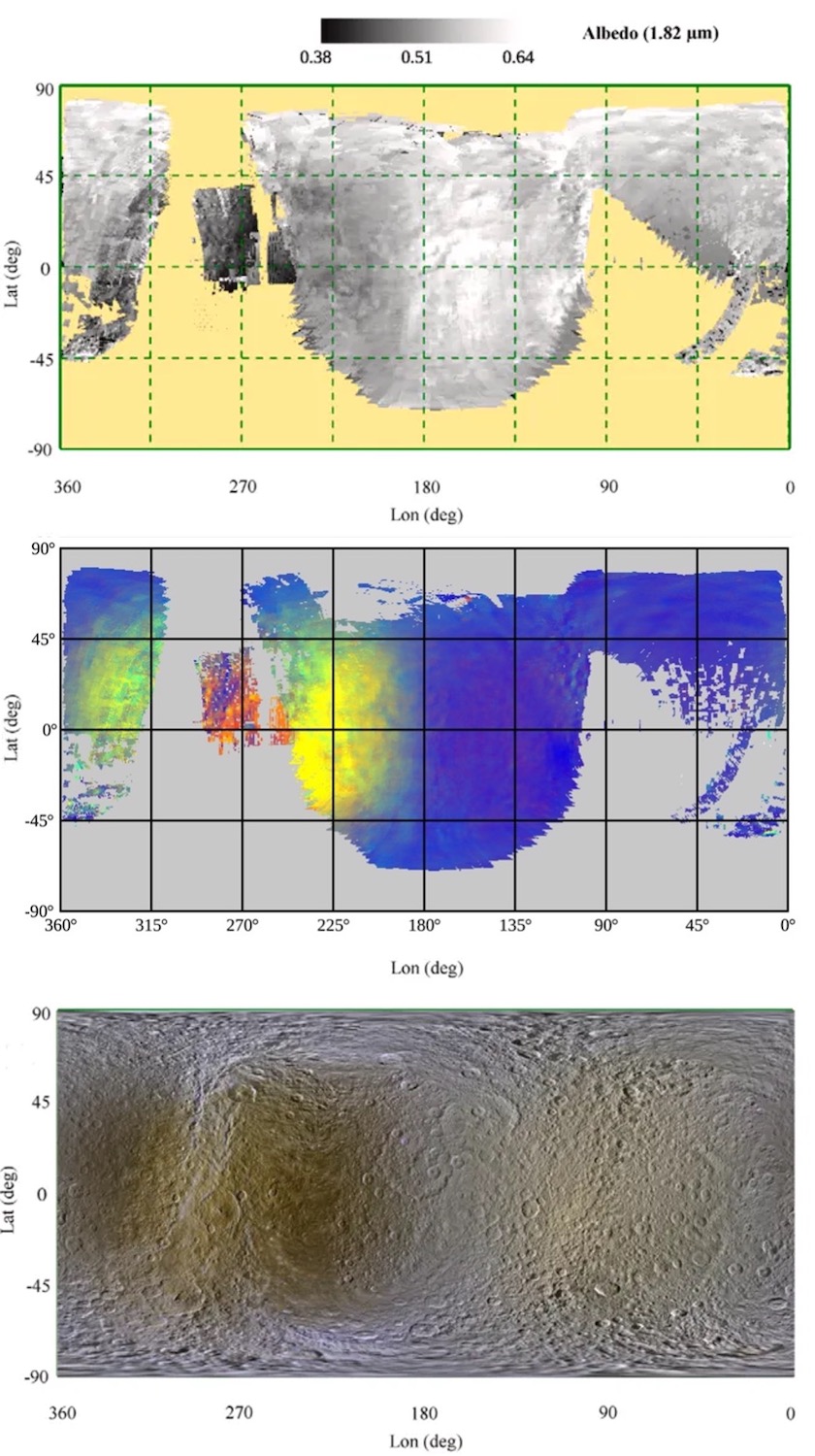
Figure 2. Top panel: 1.82-µm equigonal albedo map of Rhea in cylindrical projection [7]. Middle panel: RGB compositional map of Rhea (red: tholin abundance; green: carbon abundance; blue: water ice abundance). Bottom panel: Cassini Imaging Science Subsystem global color (IR, Green, UV) map (PIA18438, image credit P. Schenck, NASA/JPL-Caltech/Space Science Institute/Lunar and Planetary Institute).
Acknowledgments: This work is supported by the INAF data analysis grant "MId-sized Saturnian icy Satellites Investigation by Spectral modeling" (MISSIS).
References: [1] Brown et al., 2004. SSRv 115, 111–168. [2] Filacchione et al., 2012. Icarus 220, 1064–1096. [3] Filacchione et al., 2013. ApJ 766. [4] Hendrix, et al., 2018. Icarus 300, 103–114. [5] Stephan et al., 2016. Icarus 274, 1–22. [6] Scipioni et al., 2017. Icarus 290, 183–200. [7] Filacchione et al., 2022. Icarus 375. [8] Hapke, B., 2012. Theory of reflectance and emittance spectroscopy (Cambridge Univ. Press). [9] Clark et al., 2008. Icarus 193, 372–386. [10] Ciarniello et al., 2011. Icarus 214, 541–555. [11] Ciarniello et al., 2019. Icarus 317, 242-265. [12] Hendrix et al., 2018. Icarus 300, 103–114.