The possibility and feasibility of future drone-based shallow subsurface GPR radar survey for Mars have been examined. Various observations indicate shallower features are present on Mars, could be surveyed ideally from a low altitude flying airborne GPR with survey around 100 MHz transmitted frequency. Although there are uncertainties especially related to the role of iron-oxides – but in general better and deeper penetration is expected for the radar signals than it is characteristic on the Earth. The proposed instrument will be able to explore discontinuities in the underground to measure thickness, volume and stratigraphic sequence.
Introduction
This project aims to evaluate the feasibility and realization of a small mass drone based GPR survey on Mars to support next missions. Range of scientific questions on tectonics, volcanism, climate changes, water and ice could be answered of partly clarified with better knowledge on the shallow subsurface of Mars – all are ideal targets for GPR analysis. The discoveries of shallow subsurface ice on Mars (Dundas et al. 2021, Morgan et al. 2021, Mouginot et al. 2012, Schiff and Gregg 2022), and the mapping of these deposits (Putzing et al. 2023, Morgan et al. 2021), make shallow subsurface region especially important recently. While the top surface is influenced by UV- plus charged particle irradiation, as well as heavily oxidized, the few meters deep region is not influenced by these effected, thus ideal for the acquisition of astrobiology relevant samples.
Radar observations are important for Mars and based on the former MARSIS (Jordan et al. 2009, Picardi et al. 2004, Seu et al. 2007) and SHARAD instruments used from orbit, the RIMFAX instrument onboard the Perseverance and RoPeR instrument onboard Zhurong rovers could provide a range of such discoveries. Internal structure of the polar caps (Jawin et al. 2022), thickness so some depositional units (Li et al. 2022), occurrence of buried ice masses (Nerozzi and Holt 2019) have already demonstrated the success of GPR-like technology for Mars.
Targets on Mars
it is expected that the shallow subsurface consists mainly porous basaltic materials together with various salts beside sedoments. The porous voids if filled by brine or ice could increase electrical conductivity and cause strong radar reflection (Stillman et al. 2022). Porosity exhibits an elevated value at shallow depths in the regolith. Using InSight mission pores are expected to be closed around 9 km depth (Gyalay and Nimmo 2022), and close to the surface the porosity might be up to about 50-60% (Grott et al. 2022). Brines are expected also on Mars, permittivity of salty solutions depends on temperature. Brine mixtures in JSC Mars-1 regolith simulant also show a range of permittivity values depending on temperature and concentration (Kobayashi et al. 2023).
Considering the morphology of target features, surveying about HiRISE images the existence of subsurface structures in the top 10-20 m layer. Some examples are indicated in Figure 1.
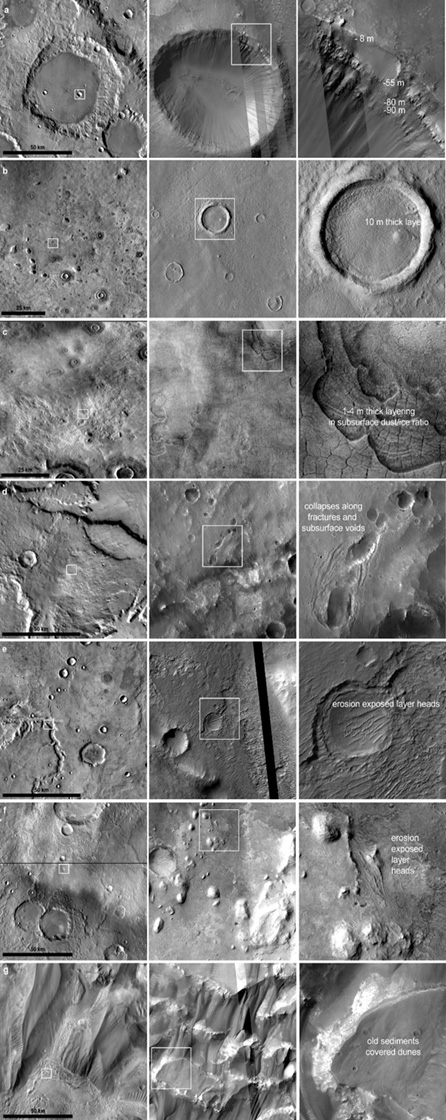
Figure 1: Surface exposed shallow subsurface structures on HiRISE images. First column shows 100 km diameter terrain using THEMIS images for context, second column shows about 4 km diameter part of the HiRISE images, while third column shows 1x1 km magnified section of the images.
Required technical parameters
Aiming the top 1-40 m of the Martian regolith the wavelength range 100-200 MHz is idel. The spatial resolution is expected in the range of meters horizontally (controlled by the pulse frequency and UAV (drone) velocity). Duricrust, hydration-related mineral filled voids and air-filled voids are expected (Spray 2004). Earth based studies showed iron-oxide lower wave velocity, bound water content also has an effect, controlling permittivity (Van Dam et al. 2002).
Identification of bulk liquids water might be moderately straightforward (Wu et al. 2019), as it absorbs the radar signal – but could be rare or absent on Mars today. Thus radar signal could penetrate deeper on Mars than in the case of the Earth. The elevated abundance of iron containing minerals on Mars could potentially affect the interpretability of GPR signal by signal attenuation caused of magnetic minerals (Heggy et al. 2001) could still be too provide useful data (Pettinelli et al. 2007).
Considering such a “FlyRadar” mission with the technical capabilities listed above, an airborne shallow subsurface radar would provide range of new information on ice content of indurated dunes, internal layering of fluvial deposits, mid- and high latitude ice, former crater lake sediments, lava caves, and subsurface tectonic structures and volcanic plumbing systems, or source region of volcano-ice interaction.
Conclusions
Drone based GPR survey on Mars could explore a poorly known domains: large (10-100 km) size area at 1-50 m shallow subsurface, what is and was in close contact with the atmosphere and volatile circulation. Enhanced mobility and accessibility even at rough, steep and dangerous areas are supported by drone-based survey. Rapid mapping of large areal in a short period with repeated observations at different daily and seasonal cycles would provide unique results.
For drones there are limitations on the size and weight of the antenna that can be carried, 100 MHz looks to be ideal. Additionally, at these wavelengths, the attenuation of the electromagnetic waves in the Martian subsurface is still acceptable, based on the attenuation at frequencies of SHARAD and MARSIS.
Acknowledgement
This work was supported by the FlyRadar EU project (No 101007973). The support from Internal fund from Thales Alenia Space Italia is also acknowledged.
References
Dundas et al. 2021. JGR 126, e06617.
Grott et al. 2021. 52nd LPSC #1237.
Gyalay and Nimmo 2022. 53rd LPSC #1633
Heggy et al. 2001. Icarus 154, 244–257.
Jawin et al. 2022. GRL 49, e99896.
Jordan et al. 2009. PSS 57, 1975–1986.
Kobayashi et al. 2023. Earth, Planets and Space 75, id.8.
Morgan et al. 2021. Nat Astron 5, 230–236.
Mouginot et al. 2012. GRL 39, L02202.
Nerozzi and Holt 2019. GRL 46, 7278-7286.
Pettinelli et al. 2007. IEEE Trans. 45, 1271-1281.
Schiff and Gregg 2022. Icarus 383, 115063.
Spray 2004. AGU, id.P33B-05
Picardi G. et al., in Mars Express, ed. by Wilson and Chicarro. SP-1240 (ESA, Noordwijk, 2004), 51–69.
Putzig 2017. 5th International Planetary Dunes Workshop #3054.
Seu et l. 2007. JGR 112(E5), E002745.
Stillman et al. 2022. JGR 127, E007398.
Van Dam et al. 2002. Geophysics 67(2):536-545.