Multiple terms: term1 term2
red apples
returns results with all terms like:
Fructose levels in red and green apples
Precise match in quotes: "term1 term2"
"red apples"
returns results matching exactly like:
Anthocyanin biosynthesis in red apples
Exclude a term with -: term1 -term2
apples -red
returns results containing apples but not red:
Malic acid in green apples
hits for "" in
Network problems
Server timeout
Invalid search term
Too many requests
TP2
Session assets
Introduction: The response of a planet to loading is intimately linked to its interior structure. On Earth, studying the time-variable response of the lithosphere to the growth and decay of large ice sheets, or glacial isostatic adjustment (GIA), has been the standard approach to constrain the mantle structure [1]. However, such models have rarely been applied to the other terrestrial planets because of a lack of observational data [2].
Mars harbors two geologically young (<100 Ma) and large (~1000 km across) polar ice caps [3], which represent the only million-year-old surface features that can induce measurable surface deformations. Radar sounders have mapped these ice caps [4,5] and the lack of lithospheric flexure beneath the north polar cap (Fig. 1a,b) was used to constrain the interior of the planet to be cold with a stiff elastic lithosphere [4,5,7]. While the results of these studies are used to constrain the present-day thermal state of Mars [8], geodynamic models [9] struggle to explain the stiff lithosphere inferred at the north pole and, at the same time, young volcanism [10] and ongoing plume activity [11] (Fig. 1c).
Importantly, previous analyses have assumed the polar deformation to be at equilibrium, which is only valid if the time elapsed since the ice cap formed is greater than the time required for viscous adjustments. Previous calculations suggested that viscoelastic relaxation could allow thinner lithospheres and higher heat flows beneath the northern cap [4,5]. However, these estimations were based on simplistic constant mantle viscosity interiors, and only examined a single wavelength to represent the load, thereby providing limited insights into the potential effects of viscoelastic relaxation. Here, we investigate GIA on Mars in light of newly acquired InSight constraints on the planet’s interior structure [8–12]. Viscoelastic simulations of the polar deformations are coupled with thermal evolution models that account for InSight seismic measurements and observational constraints on recent volcanic activity [9–11].
Methods: We have run a large suite of 3-D thermal evolution models using interior structure parameters constrained by InSight [9]. We test crustal thickness models [12] and vary other key parameters such as the distribution of radioactive heat sources [13]. The occurrence of present-day melting in these simulations is used to select acceptable models. In total, 17 models with a wide range of crustal structures are selected (Fig. 1). None of these models match the results from [4,5], and in absence of external constraints, all these models are equally possible.
To explore the extent of relaxation beneath the north polar cap, Love numbers are computed using ALMA [14]. Therein, the momentum equation of an incompressible Maxwell viscoelastic planet is solved applying a propagator method. Love numbers are calculated up to Legendre degree 50, which is sufficient to resolve the polar cap loading (~degree 8, ~1250 km wavelength).
For each of the 17 models, the predicted internal structure beneath the northern cap (>60°N) is discretized into 68 constant-thickness layers with given viscosity, density, and rigidity, from the core to the surface (Fig. 2). The time evolution of the deformation for these models is predicted using ALMA and the polar cap load potential [5,7]. Our model assumes that the ice cap formed instantly at a given time in Martian history. We also estimate the rate of deformation and the associated time-variable degree-2 zonal gravity coefficient (J2). A model is deemed acceptable if the predicted viscoelastic deformation matches radar observations [5].
Results and conclusions: The present-day interior structure beneath the northern cap shows mantle viscosities ranging from 1021 to 1024 Pa s (Fig. 2). Given the large interior viscosity, the equilibrium response of the interior to loading is achieved over long-timescales (>100 Myr). The north polar cap is young (<10 Ma, [15]), which implies that GIA is presently occurring on Mars. As a result, the elastic lithosphere thickness estimated in [4,5] are overestimated. In particular, some geodynamic models that were deemed unsuccessful because of their mismatch to the north polar elastic thickness are now acceptable (Fig. 1-2).
Only a subset of models predicts small polar deformations consistent with radar observations [4,5], and these are characterized by a thick crust (≥50 km on average), cold lithosphere (elastic thickness >150 km), and large mantle viscosity (>1022 Pa s). A thick crust is consistent with the independent reassessment of InSight seismic data [16]. Models with a higher crustal heat production cool faster, have higher mantle viscosities, slower interior response to loading and thus better fit radar observations. For accepted models, GIA in the polar regions show rates >10-3 mm/year and strain rates >10-20 s-1.
Our analysis further constrains the bulk of the northern cap to have formed no more than 7 Myr ago, which is in remarkable agreement with independent Global Climate Model [15]. Models with a linear ice cap formation would imply even younger ages. If the mantle viscosity were precisely known, our framework could be used to place constraints on the formation history of the ice cap.
GIA affects the long-wavelength gravity field. Over the last 20 years, J2 may have varied by up to 10-11 m s-2. Together, our results implies that GIA could be measured from precise laser ranging or with a dedicated GRAIL-like gravity mission to Mars [17,18]. Alternatively, reanalyses and extended collection of radio tracking data could enable discerning a trend in the gravity field.
References: [1] Peltier W. R. (1974). [2] Greve R., et al. (2003) [3] Byrne S. (2009). [4] Phillips R. et al. (2008). [5] Broquet A. et al. (2020). [6] Broquet A. (2020) AB-Ares/Te_HF_Conversion [7] Broquet A. et al. (2021) JGR:Planets. [8] Khan A. et al. (2021). [9] Plesa A.-C. et al. (2022). [10] Voigt J. R. C. et al. (2023). [11] Broquet A. & Andrews-Hanna J. C. (2023). [12] Wieczorek M. A. et al. (2022). [13] Hahn B. C. et al. (2011). [14] Spada G. (2008). [15] Levrard B. et al. (2007). [16] Kim D. et al. (2023). [17] Genova A. (2020). [18] Wörner L. et al. (2023).
How to cite: Broquet, A., Plesa, A.-C., Breuer, D., Wieczorek, M. A., Root, B. C., Klemann, V., Genova, A., and Andrews-Hanna, J. C.: Glacial isostatic adjustment on Mars, Europlanet Science Congress 2024, Berlin, Germany, 8–13 Sep 2024, EPSC2024-847, https://doi.org/10.5194/epsc2024-847, 2024.
NASA’s InSight mission has brought new information about the Martian lithosphere (Banerdt et al. 2020), which warrants a re-analysis of the support of the crustal and sub-crustal masses. Furthermore, the discovery of a possible mantle plume underneath the region south of Elysium Mons (Broquet et al. 2023), gives evidence for recent magmatic activity underneath the crust of Mars causing dynamic support to volcanic structures.
The goal of this study is to combine a global flexural model combined with mantle convection modelling to study the different uncertainties in the geophysical parameters that dictate crustal (short-scale) and mantle (large-scale) mass anomalies. After conducting spectral analysis on the gravitational signal of we found that the Martian lithosphere can be best modelled by an elastic thin shell with the following physical parameters: crustal thickness of 60 ±10 km, crustal density of 3050 ±50 kg/m3, mantle density of 3550 ±100 kg/m3, and the elastic thickness (Te) is found to be 80 ±5 km.
Figure 1: Crustal density from short scale gravity anomalies inversion. The flexural model is not able to represent to perfect isostasy ate Hellas Basin (and assumed present also at Utopia basin). Therefore, high crustal density is seen reflecting a shallower crust-mantle interface. Isidis and Argyle basin also show high mass regions that could be interpreted as shallow crust-mantle boundary or magmatic intrusions in the crust. Other high mass features are related to volcanoes and large impact craters. Finally, the northern polar planes show also anomalous high mass regions.
By using this spectral analysis, we were able to isolate the remaining long-wavelength signal that cannot be realistically modelled by a flexure model. The location of the residual anomaly correlates with the Tharsis Rise, which suggests active large-scale dynamic support of the volcanic region. A negative mass anomaly in the mantle underneath the Tharsis Region explains the remaining gravity residual. This anomaly could be interpreted as underplating of the lithosphere, a phase transition anomaly at 1000 km depth, or an rising mantle plume. Could mantle convection is still be active in Mars explaining the relatively young geologic surface volcanism?
The remaining short scale gravity residuals give insight in Martian crustal density distributions (Figure 1). Flexure models cannot account for these structures as the lithosphere is strong enough to reduce/negate any topographic signature. Especially in the northern polar plains several buried mass anomalies have been detected (Figure 2). The nature of these anomalies is unclear, as they could be interpreted with a volcanic origin, impact related structures, or tectonic orogeny that would all be buried be the sedimentary layer that is observed on the surface of the northern hemisphere. If these structures are interpreted as impact craters this would suggest of an older crustal age of the northern hemisphere of Mars than is now considered. New gravity satellite missions towards Mars are needed to uncover the nature of these subsurface mass anomalies (Wörner et al 2023, Genova et al 2020).
Figure 2: Contours of the high mass anomalies in the nortern polar plains. These high mass anomalies seem to have no topograohic signature. Topography is shown in the colorscale and taken from the MOLA dataset.
References:
- Banerdt, W. B., Smrekar, S. E., Banfield, D., Giardini, D., Golombek, M., Johnson, C. L., … Wieczorek, M. (2020, March). Initial results from the InSight mission on Mars. Nature Geoscience, 13 (3), 183–189.
- Broquet, A., & Andrews-Hanna, J. C. (2023). Geophysical evidence for an active mantle plume underneath Elysium Planitia on Mars. Nature Astronomy, 7 (2),160–169.
- Genova, A. (2020), ORACLE: A mission concept to study Mars’ climate, surface and interior, Acta Astronautica, 166, 317-329.
- Wörner, L., B.C. Root, P. Bouyer, C. Braxmaier, D. Dirkx, J. Encarnação, E. Hauber, H. Hussmann, Ö. Karatekin, A. Koch, L. Kumanchik, F. Migliaccio, M. Reguzzoni, B. Ritter, M. Schilling, C. Schubert, C. Thieulot, W.v. Klitzing, O. Witasse (2023), MaQuIs—Concept for a Mars Quantum Gravity Mission, Planetary and Space Science, 239, 105800.
How to cite: Root, B., Alkahal, R., Qin, W., and Thieulot, C.: Exploration of high mass subsurface structures in the northern hemisphere with joint flexure and mantle convection modelling of the Martian gravity field , Europlanet Science Congress 2024, Berlin, Germany, 8–13 Sep 2024, EPSC2024-730, https://doi.org/10.5194/epsc2024-730, 2024.
Impact basins, the largest type of impact craters, form during the early stages of planetary evolution in the first several hundred million years following planetary accretion. Their size and morphology are mainly controlled by impact speed, impactor mass and geological properties of the target at the time of impact. Significant progress has been made in comprehending impact basins in recent years, with most efforts aimed at the Moon, where basins are best preserved. However, our comprehension of Mars basins remains comparatively limited. To fill this gap, we used numerical simulations to study the formation of impact basins on Mars at different geological epochs and regions.
We simulated the formation of impact basins using iSALE-2D, a multi-material, multi-rheology shock physics code that can simulate impacts in geological materials [www.isale-code.de]. We considered vertical impacts of dunite impactors travelling at 10 km/s on a target composed of a basaltic crust and a dunitic mantle with a surface temperature of 213 K. Projectile sizes varied from 20 to 400 km in diameter. The target was considered flat for simulations involving impactors smaller than 200 km; larger impacts were evaluated using both flat and spherical target geometry. We adopted three temperature profiles representing the planet's thermal state at 4.4 Ga (soon after the crust formed, when it was relatively hot), 4.0 Ga (an intermediate state) and 3.5 Ga (when the crust was comparatively colder). Two crustal thicknesses were considered, 47 and 91 km, corresponding to the average values of the martian lowlands and highlands.
Our simulations were presented as cross-sections of half craters showing two distinct layers, crust and mantle. We enabled material tracing to follow the material movement. We measured the spacing of rings indicated by tracer displacements and compared their position to the overall morphology of the simulated basins. We observed the formation of a listric normal fault at the main rim, crosscutting the crust and mantle to depths comparable to the maximum excavation depth. This structure, referred to as "main fault", was observed across all basin sizes considered in this study, and thus was considered as a suitable marker for the final basin size. In sufficiently large basins, we observed an outer ring defined by a planar normal fault crosscutting crust and mantle to comparatively larger depths. This structure, referred to as "outer ring fault", was associated with a topographical high and a zone of localised crustal thinning, forming crustal blocks similar to the ones observed in regions of extensional tectonics.
The spacing of rings in our simulated basins is similar to the reported spacing of rings in lunar basins. On average, the inner and outer rings are positioned at 0.46 and 1.41 times the final basin radius respectively (Figure 1). This spacing is controlled by age and crustal thickness. Rings of basins formed in older basins or hotter crust tend to be positioned closer to the impact point than those formed in younger basins or colder crust. Crustal thickness excerpt a similar effect, especially on the position of outer rings. Outer rings of basins formed in a thin crust are ~14% closer to the impact point than outer rings of basins formed in a thick crust.
Our results have implications for the formation and evolution of impact basins on Mars, helping to deepen our understanding of basin’s inner structure and multi-ring basin formation. Moreover, our results can be used to constrain the thermal state and crustal structure on Mars at the time of basin formation by comparing our findings with the observed morphology of Martian basins. This provides important insights to the bombardment history of Mars and to our knowledge of large impacts in the early Solar System.
Figure 1 - Relative ring spacing of the inner (rinn) and outer (rout) rings measured from 60 numerical simulations of impact basins on Mars created by projectiles from 20 to 200 km in diameter. The plots present, from top to bottom, the complete dataset, and simulations for 3.5, 4.0 and 4.4 Ga. Inner ring ratios for a thin and thick crust are presented in orange and blue respectively; outer ring ratios for a thin and thick crust are presented in red and green respectively. In the rare cases more than one outer ring was present, only the inner most radii were recorded.
How to cite: Branco, H., Miljkovic, K., and Plesa, A.-C.: Ring formation in impact basins on Mars, Europlanet Science Congress 2024, Berlin, Germany, 8–13 Sep 2024, EPSC2024-288, https://doi.org/10.5194/epsc2024-288, 2024.
Introduction: Since the 60’s, extensive surveys with orbiters have produced large quantity of data on planet Mars, including very high resolution products (e.g. [1-2]) that allow precise studies of the surface geomorphology. Quantitative approaches such as morphometry have been broadly used on orbital imagery to produce impact craters scaling laws [3-5]. These laws are needed to quantify erosion (e.g. [6]), and by extension to better understand the surface processes that occur on the red planet. However, only one study has focused on crater diameters below 1 km [5] and scaling laws for diameters below 50 m have not been investigated yet. In our study, we counted the craters and measured their depths and diameters on landslide deposits located in Valles Marineris [7]. We produced a scaling law of depth d as a function of diameter D for craters below 50 m.
Methods: We used High Resolution Imaging Science Experiment (HiRISE, [1]) products to count all the craters in a specific area. We focused on ESP_024255_1750, a HiRISE image and the associated Digital Elevation Models (DEM, [2]), which both have a resolution of 0.5 m/pixel. We mapped the landslide deposits, which are restricted to the northern part of the image, at the base of the canyon walls. The data have been studied with Geographical Information System (GIS) Arcmap 10.8. We first counted all the craters at the surface and made diameters measurement using the HiRISE image. Then, depths have been measured following our handmade model through the ModelBuilder tool. The model fitted a local surface for each crater from elevations encompassing the crater rims, on a 3 m buffer area. We finally subtracted the elevations from the local surface and the DEM to obtain the crater depth.
Results and discussion: We counted 32128 craters of all diameters on ESP_024255_01750 within the landslide region. We reduced this count to keep all the craters with D > 10 m and excluded the negative depths that are due to DEM vertical uncertainties. This yielded a final count of 1252 craters. We fitted the freshest craters to get the morphometry under the form d = cDα following the methods from Boyce and Garbeil, 2007 [3] (figure 1). This produced the following expression:
d = [0.137±0.290] D[0.862±0.419] ,
where d and D are depths and diameters in meters. Parameter α is slightly lower than the value found in Watters et al., 2015 (α = 0.205±0.012, [5]), highlighting that craters are shallower in this range of diameters. Parameter c has an intermediate value between those from Watters et al., 2015 (c = 1.012±0.009 [5]) and Garvin et al., 2003 (c = 0.81 [4]). These results have to be compared with our previous results in which we found a higher value for c (c = 1.014, Millot et al., 2024, conference paper submitted to the 10th International Conference on Mars [8]) on Interior Layered Deposits (ILDs, e.g. [9]), light-toned sedimentary rocks that are known to be easily erodible [6]. These differences highlight the impact of terrains on the scaling laws. Finally, parameters c and α have high uncertainties due to the small area involved in the count: we expect these uncertainties to decrease if we include more landslide areas in the counting. Given the very large number of craters comprised within a single region restricted to the northern part of a HiRISE image, next studies will involve machine learning algorithms (e.g. [10]) to perform automatic segmentation and mapping of the impact craters. These tools will be mandatory to refine our results over larger regions, and reconcile local and global scale analyses of the Martian surface.
Figure 1: Log-log plot of depth d versus diameter D for the crater dataset on the landslide. Plain black points are included in the linear regression (red dashed line). The gray points are other craters from the dataset. The scaling law is displayed under the logarithmic form, with r2 value to quantify the regression robustness.
References: [1] McEwen A. S. et al. (2007) JGR: Planets, 112, E5. [2] Kirk R. L. (2008) JGR: Planets, 113, E3. [3] Boyce J. M. and Garbeil H. (2007) Geophysical Research Letters, 34, 16. [4] Garvin J. B. et al. (2003) Sixth International Conference on Mars, Abstract #3277. [5] Watters W. A. et al. (2015) JGR: Planets, 120, 2, 226-254. [6] Kite E. S. and David P. M. (2017) Icarus, 286, 212-222. [7] Quantin C. et al., (2004) Icarus, 172, 2, 555-572. [8] Millot C. et al. (2003) Submitted to Tenth International Conference on Mars, Abstract #3355 [9] Mangold N. et al. (2008) Icarus, 194, 2, 519-543. [10] C. Lee (2019), PSS, 170, 16-28.
How to cite: Millot, C., Quantin-Nataf, C., Dehouck, E., Volat, M., and Torres, I.: Morphometric Laws for Small Martian Craters (D < 50 m): a Case Study on Landslide Deposits, Europlanet Science Congress 2024, Berlin, Germany, 8–13 Sep 2024, EPSC2024-844, https://doi.org/10.5194/epsc2024-844, 2024.
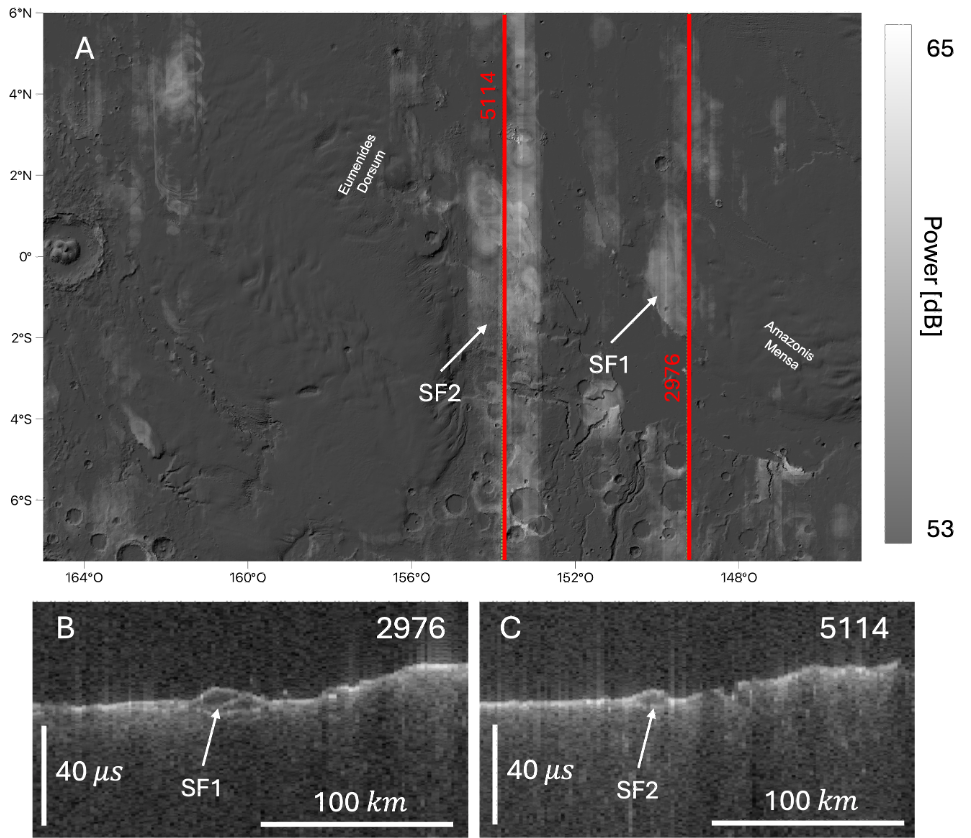
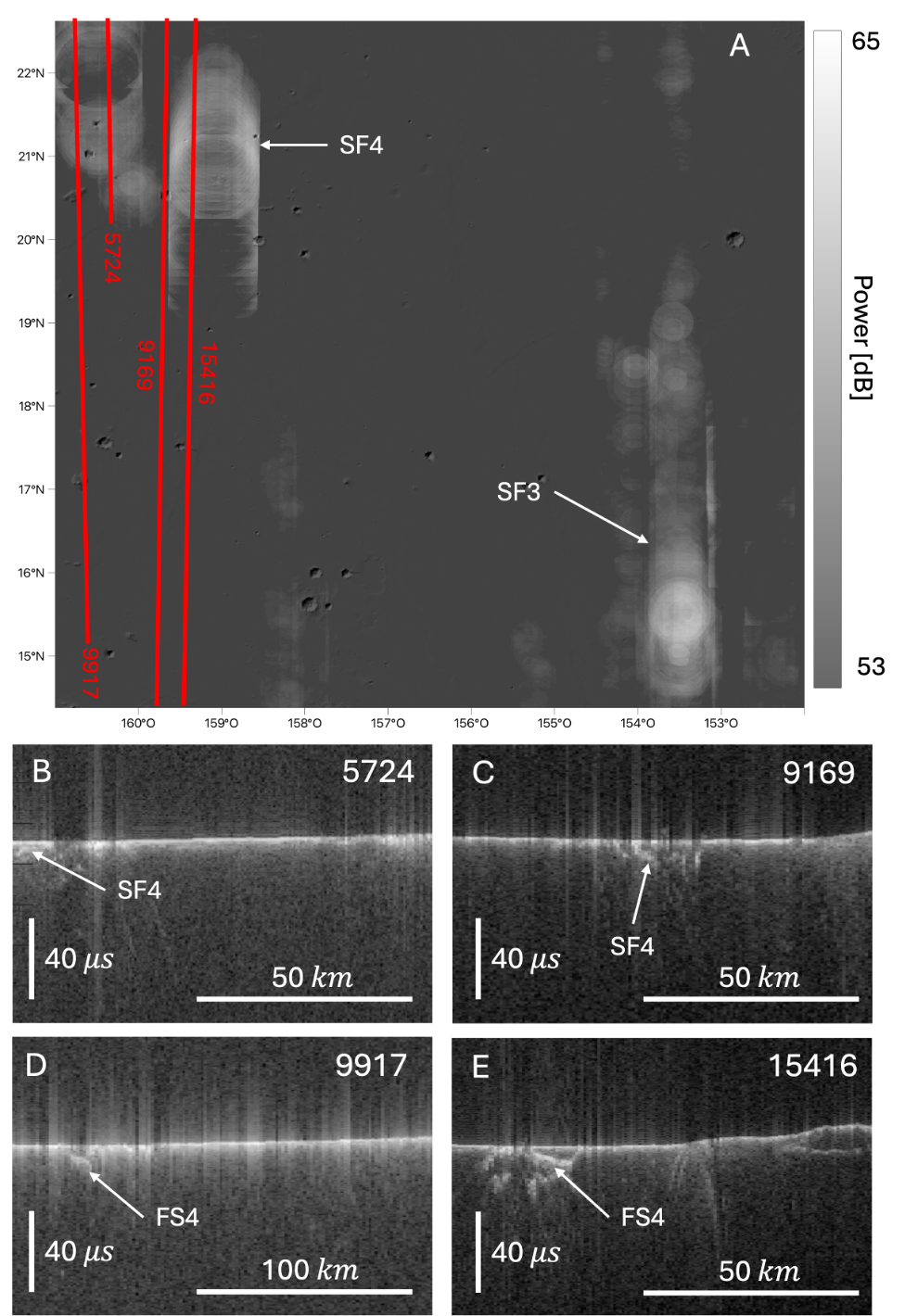
How to cite: Hoyo García, M., Bruzzone, L., and Bovolo, F.: Enabling large-scale detection of subsurface features on Mars by their radar mapping, Europlanet Science Congress 2024, Berlin, Germany, 8–13 Sep 2024, EPSC2024-61, https://doi.org/10.5194/epsc2024-61, 2024.
Introduction
China’s first Mars exploration mission, Tianwen-1, was successfully launched on 23rd July 2020. The mission included an orbiter, a lander, and the Zhurong rover. Zhurong landed on Mars in the southern part of Utopia Planitia (109.925°E, 25.066°N) on 15th May 2021 and then conducted unprecedented in-situ observations with several instruments, including a ground-penetrating radar (GPR) called Rover Subsurface Penetrating Radar (RoSPR) (Liu et al., 2023). The radar (Fig. 1) aimed at probing the subsurface at the Zhurong landing site and searching for buried water ice. Utopia Planitia is well known for showing different ice-related landforms (Séjourné et al., 2019). To achieve this goal, RoSPR was equipped with two channels: a high-frequency channel providing high-resolution (5 cm, when permittivity is 3) full-polarization radargram of the shallow subsurface (first 10 meters) and a low-frequency channel capable of penetrating the Martian subsurface down to a depth of ~100 meters with a 1-meter vertical resolution.
In-situ GPR observation can reveal the structure and permittivity distribution along the cross-section drawn by the rover’s traverse. The subsurface structure is interpreted from the radar profile, which intuitively shows the location of subsurface reflectors, such as buried craters, aeolian deposits, and icy beds. Permittivity is a complex parameter describing the electromagnetic properties of materials. It mainly depends on composition and porosity. Its real part and imaginary part respectively control the wave velocity and signal attenuation rate in the Martian subsurface.
Data and Methods
We use all the 5893 dual-channel radar traces collected by RoSPR during the 325 solar days on Mars. The traverse of the radar is shown in Fig. 2. Those observations covered ~1840 meters with an elevation variation of ~10 meters from the Zhurong landing site southward. Data from ground experiments of RoSPR on glaciers on Earth are also used to provide a comparison between the RoSPR data acquired on different sites.
In addition to conventional RoSPR data treatments, advanced data processing methods are deployed to better reveal subsurface structure. In particular, we adopt a background matrix method (Rashed and Harbi, 2014) which statistically removes the systematic clutters. Blind deconvolution methods of the radar B-scan are also tested to address and eliminate the ringing effect that commonly exists. In addition, the bandwidth extrapolation technique designed for range resolution enhancement (Oudart et al., 2021) will be applied to further provide clarity among different buried objects.
Preliminary Results and Ongoing Work
To estimate the Martian soil permittivity, we applied a hyperbola fitting method which accounts for the antenna’s height (Wang et al. 2021, Oudart et al., 2022), and a centroid frequency shift method (Quan et Harris, 1997) which uses the real-time spectrum of dual-channel RoSPR data to maximize the accuracy (Fig. 3). Loss tangent estimation also reveals upper bounds of ~0.008 and ~0.022 for the first 10 meters and 40 meters, respectively.
The low-frequency B-scan, as shown in Fig. 4, reveals buried structures and indistinct reflectors in deeper regions. The variation of the B-scan and loss tangent value along the depth implies varied composition at the landing site. The ongoing work focuses on refining data processing techniques to improve the clarity of subsurface imaging and provide robust interpretation and geological analysis.
References
[1] S. Liu et al., “Data Pre-Processing and Signal Analysis of Tianwen-1 Rover Penetrating Radar,” Remote Sensing, vol. 15, no. 4, p. 966, Feb. 2023, doi: 10.3390/rs15040966.
[2] N. Oudart et al., “Range resolution enhancement of WISDOM/ExoMars radar soundings by the Bandwidth Extrapolation technique: Validation and application to field campaign measurements,” Planetary and Space Science, vol. 197, p. 105173, Mar. 2021, doi: 10.1016/j.pss.2021.105173.
[3] N. Oudart et al., “Retrieval of the ground dielectric permittivity by planetary GPR accommodated on a rover: Application to the estimation of the reflectors’ depth by the WISDOM/ExoMars radar,” Planetary and Space Science, vol. 224, p. 105606, Dec. 2022, doi: 10.1016/j.pss.2022.105606.
[4] Y. Quan and J. M. Harris, “Seismic attenuation tomography using the frequency shift method,” GEOPHYSICS, vol. 62, no. 3, pp. 895–905, May 1997, doi: 10.1190/1.1444197.
[5] M. Rashed and H. Harbi, “Background matrix subtraction (BMS): A novel background removal algorithm for GPR data,” Journal of Applied Geophysics, vol. 106, pp. 154–163, Jul. 2014, doi: 10.1016/j.jappgeo.2014.04.022.
[6]A. Séjourné et al., “Grid Mapping the Northern Plains of Mars: Using Morphotype and Distribution of Ice-Related Landforms to Understand Multiple Ice-Rich Deposits in Utopia Planitia,” Journal of Geophysical Research: Planets, vol. 124, no. 2, pp. 483–503, Feb. 2019, doi: 10.1029/2018JE005665.
[7] R. Wang et al., “A Novel Approach for Permittivity Estimation of Lunar Regolith Using the Lunar Penetrating Radar Onboard Chang’E-4 Rover,” Remote Sensing, vol. 13, no. 18, p. 3679, Sep. 2021, doi: 10.3390/rs13183679.
Fig. 1 The dual-channel RoSPR onboard Zhurong rover. The low-frequency monopole antennas are mounted in the front of the rover. The high-frequency Vivaldi antennas are tied to the rover’s body.
Fig. 2 The traverse of Zhurong rover and the elevation at the landing site.
Fig. 3 The loss tangent estimation via RoSPR low-frequency data. The figure demonstrates the centroid frequency decay versus time delay relationship, calculated from 160 traces of Low-frequency channel data ranging from ~300 to ~550 ns.
Fig. 4 RoSPR low-frequency B-scan section from sol 112 to sol 288.
How to cite: Zhang, Z., Schmidt, F., Su, Y., Oudart, N., Le Gall, A., Saintenoy, A., Brighi, E., Ciarletti, V., Léger, E., Costard, F., and Séjourné, A.: Analysis of the Martian subsurface from the observation of the Rover Subsurface Penetrating Radar (RoSPR) onboard Tianwen-1, Europlanet Science Congress 2024, Berlin, Germany, 8–13 Sep 2024, EPSC2024-441, https://doi.org/10.5194/epsc2024-441, 2024.
Introduction: NASA's Perseverance Rover is exploring the Jezero Crater carrying the RIMFAX ground penetrating radar. RIMFAX has acquired a continuous subsurface radar image at 10 cm intervals along the rover’s > 25 km long ground track across the crater floor and fan, probing depths of >30 m below the rover, Figure 1. RIMFAX provides subsurface context for better understanding the depositional environments of the geological units that the rover has examined on the crater floor and the fan thus far. The Mars 2020 mission objectives are to seek signs of ancient life on Mars and cache a set of samples for possible return to Earth by a follow-on mission, [1]. An important part of the mission is to document the geological settings the cached samples are collected from. The Radar Imager of the Mars’ Subsurface Experiment is a gated FMCW radar operating in the frequency band of 150 – 1200 MHz. The antenna is a slotted bow tie antenna 70 cm above the surface. The typical center frequency of the reflected signal at 10–15-meter depth is 400 Mhz. RIMFAX collects soundings in three operating modes (surface, shallow and deep) every 10 cm along the rover’s traverse path, [2].
Figure 1 Orbital context maps of the RIMFAX observations in Jezero Crater. Orbital High Resolution Imaging Science Experiment (HiRISE) color base map showing the path (pale white lines) of the Perseverance rover.
RIMFAX Radar Data: In the Margin Unit RIMFAX images several strong dipping layers that get horizontal at depth resembling clinoforms, Figure 2. The location of these reflecting layers is picked from the RIMFAX radargrams giving interface points in depth and location of the different layers.
Figure 2 Radargrams give the reflected signal where white is high reflecting amplitude and black low amplitude. The depth axis for this radargram is 35 meters.
Geological Modeling: The RIMFAX data can be used to make 3D models of the subsurface. The depth and locations of selected layers are picked from the RIMFAX Marginal Unit radargrams. From these layers, a geological model is made using GemPy, see [4] which employs a universal cokriging interpolation method, [4]. GemPy allows the user to generate complex 3D structural geological models through the interpolation of layer interfaces and orientation measurements and topography.
Figure 3 shows a 2D cross section through the GemPy model. Figure 4 shows a map view of the model, indicating locations of outcropping layer boundaries. Figure 5 shows a 3D view of the subsurface layers in relation to surface topography.
Figure 3. 2D cross section through the GemPy model from West to East. Non-shaded layers are truncated by surface topography.
Figure 4. GemPy model reconstructed geologic map based on the extrapolation of picked layer depths and locations in the RIMFAX radargrams in the Margin Unit (dots).
Figure 5. 3D geological model reconstructed from RIMFAX data showing the subsurface dipping layers and where they crop out on the surface.
Discussion: The large-scale subsurface structures revealed by the RIMFAX in the Marginal Unit are reminiscent of deltaic clinoforms. Figure 4 shows that Marginal Unit layers are cropping out in complicated patterns that are not apparent in orbital images or topographic maps. Deeper eroded layers are predicted to be cropping out in the wall of Neretva Vallis. Using RIMFAX radar data in conjunction with geological modelling is a powerful tool for studying subsurface structures on Mars.
Acknowledgments: The data used in this work are available at the NASA PDS Geosciences Node (https://doi.org/10.17189/1522644). This work was supported by the Research Council of Norway, grant no. 309835.
References:
[1] Farley et. al. (2021) Space Science Review, [2] Hamran et. al. (2021) Space Science Review, [3] Hamran et. al. (2022) Science Advances, [4] de la Varga et. al. (2019) GemPy, Geosci. Model Dev., 12, 1–32
How to cite: Hamran, S.-E., Paige, D., Andersson, F., Berger, T., Cardarelli, E., Carter, L., Dypvik, H., Russell, P., Mellon, M., Nunes, D., and Plettemeier, D.: Geological Modeling of Subsurface Structures from RIMFAX Ground Penetrating Radar Observations in Jezero Crater, Mars. , Europlanet Science Congress 2024, Berlin, Germany, 8–13 Sep 2024, EPSC2024-403, https://doi.org/10.5194/epsc2024-403, 2024.
Introduction :
In planetary sciences, accessing the subsurface is essential to fully understand the geological history of a given site. GPR (Ground Penetrating Radar) offers a way to investigate the subsurface in a non-destructive manner. So far, five GPR have investigated the subsurface of the Moon and Mars from the surface. RIMFAX GPR [1] onboard the Perseverance rover of NASA’s Mars 2020 mission is still operating at Jezero crater and RoSPR [2] investigated the subsurface of Utopia Planitia along the traverse of Zhurong, the rover of the CNSA’s Tianwen-1 mission. Both RIMFAX and RoSPR are ultra-wide band GPR ; RIMFAX is a Frequency Modulated Continuous Wave radar operating from 150 to 1200 MHz while RoSPR is equipped with two channels : which respectively operate in the 15-95 MHz (low frequency or LF channel) and the 450-2150 MHz (high frequency or HF channel) ranges. The next GPR to Mars should be WISDOM [5] onboard the Rosalind Franklin rover of the ESA’s ExoMars mission [8]; it is designed to probe the subsurface at Oxia Planum at frequencies in the 0.5-3 GHz range.
Clear buried interfaces or resolvable large reflecting structures are probably the most easily interpretable features a GPR can detect in the subsurface. However, radargrams often display diffuse scattering in the subsurface volume rather than clear structures. As an example, the radargram acquired by RoSPR at Utopia Planitia on Mars points to the presence in the subsurface of two layers containing scatterers of different size or nature, but no clear interface [3]. Scattering signature was also observed in the lunar subsurface by the LPR (Lunar Penetrating Radar), and the typical size of heterogeneities in the lunar regolith has been constraint [4].
In this work we propose to apply on RoSPR and, to a lesser extent, RIMFAX data a method developed on WISDOM simulated data [5]. This method aims at statistically retrieving the typical size of buried heterogeneities or scatterers from scattered signals in radargrams. The RoSPR LF profile section selected as an example to illustrate the method is shown on Fig 1. The orange section is 280 m long and corresponds to the end of the profile published in [3] displaying unambiguous scattering down to depths of 50 meters.
Method :
We conducted FDTD simulations with the TEMSI-FD code [6], that accurately models the WISDOM antennas in order to reproduce reliable soundings over 3-D synthetic heterogeneous media as illustrated by Fig 2. We generated a statistical number of subsurface volumes to perform meaningful statistical averages. Fig. 2 upper panel shows three examples of modelled subsurfaces with different typical size of scatterers L. Volume scattering occurs for each of the three L values considered, but the strongest volume scattering is not observed at the same frequencies. In other words, the wide frequency bandwidth of WISDOM allows to study the scattering process by sub-frequency bands to estimate the typical size of the buried structures. Fig. 3 synthesizes the results obtained with a sliding frequency window analysis for L values in the range 0.7-6.2 cm. A previous study [7] performed on simulated data shows that the maximum volume scattering intensity occurs for a wavelength λ = (5.3±0.2)L regardless of the value of L. We propose to use this result on Martian radargrams displaying diffuse scattering to constraint the L value in the investigated areas.
We built spectrograms applying a Fourier Transform to have a quick and global picture of the frequency content along the profile. This can be done on selected time windows of the radargram that correspond to given depth ranges (see Fig 4). This method also reveals possible horizontal variability in the frequency content of the radar signal. The described analysis requires in the first place to compensate the variability of the instrument’s gain over the bandwidth . This is achieved by dividing the frequency domain data by the average RoSPR spectra of the subsurface of Utopia Planitia (a processing called whitening). However, in absence of proper calibration data, this correction is not perfect and some instrumental artifacts may remain (as it is the case on Fig. 4 around 47 and 84 MHz).
Results and preliminary analysis :
The result shown in Fig. 3 implies that ultra-wide band GPR data can be used to determine the size of heterogeneities within a range that depends on its frequency range of operation and of the average permittivity of the medium, as synthesized in Fig 5 for all Martian GPRs.
In Fig. 6 the spectrograms’ frequency-axes corresponding to different time windows (Fig 4) are converted into L-axes via the relation established based on simulations (Fig. 3) in order to interpret them in terms of scatterer size. The main features are indicated in Fig 6, but must be taken with cautious In particular, peaks in the volume scattering intensity can have various origins and only global maximums should be considered. Another caveat is the possible presence of several populations of scatterers of different sizes.
The difference between upper and lower maps in Fig. 6 somewhat supports the interpretation advanced by [3] with two layers characterized by smaller scatterers in the upper layer than in the lower layer). The spectrogram analysis method could put firmer constraints on their actual size distributions and horizontal scales of variability.
References :
[1] Hamran et al., Space Sci Rev (2020). https://doi.org/10.1007/s11214-020-00740-4
[2] Liu et al., Remote Sensing 2023. https://doi.org/10.3390/rs15040966
[3] Li et al., Nature (2022). https://doi.org/10.1038/s41586-022-05147-5
[4] Ding et al., A&A 2022. DOI: 10.1051/0004-6361/202142803
[5] Ciarletti et al., Astrobiology 2017. https://doi.org/10.1089/ast.2016.1532
[6] Ciarletti et al., Monthly Notices of the Royal Astronomical Society, 2017. https://doi.org/10.1093/mnras/stx3132
[7] Brighi et al., Planetary and Space Science, under review
[8] Vago et al., Astrobiology, 2017. https://doi.org/10.1089/ast.2016.1533
How to cite: Brighi, É., Oudart, N., Ciarletti, V., Le Gall, A., Zhang, Z., Su, Y., Schmidt, F., Saintenoy, A., and Hamran, S.-E.: Spectral analysis of radargrams of the Martian subsurface to constrain scatterer sizes-Application to RoSPR/Zhurong and RIMFAX/Perseverance GPR data, Europlanet Science Congress 2024, Berlin, Germany, 8–13 Sep 2024, EPSC2024-439, https://doi.org/10.5194/epsc2024-439, 2024.
The ever-changing transparency of the martian atmosphere hinders the determination of absolute surface colour from spacecraft images. While individual high-resolution images from low orbit reveal numerous colour details of the geology, the colour variation between images caused by scattering off atmospheric dust can easily be of greater magnitude. The construction of contiguous large-scale mosaics has thus required a strategy to suppress the influence of scattering, often a form of high-pass filtering, which limits their ability to convey colour variation information over distances greater than the dimensions of single images. Here we use a dedicated high altitude observation campaign with the Mars Express High Resolution Stereo Camera (HRSC), applying a novel iterative method to construct a globally self-consistent colour model. We apply the model to colour-reference a high-altitude mosaic incorporating long-range colour variation information. Using only the relative colour information internal to individual images, the influence of absolute image to image colour changes caused by scattering is minimised, while the model enables colour variations across image boundaries to be self-consistently reconstructed. The resulting mosaic shows a level of colour detail comparable to single images, while maintaining continuity of colour features over much greater distances, thereby increasing the utility of HRSC colour images in the tracing and analysis of martian surface structures.
How to cite: Michael, G., Tirsch, D., Matz, K.-D., Zuschneid, W., Hauber, E., Gwinner, K., Walter, S., Jaumann, R., Roatsch, T., Postberg, F., and Liu, J.: A global colour mosaic of Mars from Mars Express HRSC high altitude observations, Europlanet Science Congress 2024, Berlin, Germany, 8–13 Sep 2024, EPSC2024-1166, https://doi.org/10.5194/epsc2024-1166, 2024.
Introduction :
Martian H2O, CO2 and dust cycles are key processes in modern climate dynamics. The polar caps [1], as well as their icy outliers (that can take the form of convex-shaped ice mounds inside deep craters) are the current, major, exposed volatiles reservoirs. Unanswered questions remain about the mass balance of the caps. Concerning outliers, such as the ice domes of Louth and Korolev craters[2,3], the prevailing hypothesis suggests a build up by atmospheric deposition [4]. If accurate, these icy mounds could record past climate conditions while still responding to present-day Martian climate change because of their southerly location [5]. They therefore offer unique opportunities to study volatiles dynamics, with different boundary conditions than those of the north or south caps.
Research question :
Our study takes advantage of the Exomars Trace Gas Orbiter – Colour and Stereo Surface Imaging System (CaSSIS) [6] colour imaging capabilities to evaluate pluri-annual ice changes, especially water frost and dust coverage at Louth and Korolev craters ice mounds. Those changes can serve as a proxy of local dynamics, which can reveal important information about ice and dust cycles.
General methods:
CaSSIS is the high-resolution stereoscopic camera of ESA’s ExoMars Trace Gas Orbiter (TGO). It possesses 4 broad band filters across the visible range : BLU (497nm), PAN (677nm), RED (835nm) and NIR (940nm). The orbit of TGO being non-Sun-synchronous, it allows us to acquire images of the same region of interest at different seasons and local times, at 4.6 m/pixel with high a signal-to-noise ratio, and therefore allows to monitor diurnal phenomena until 74°N. The first step of the study consists of a morphological and spectral analysis of 25 Louth crater images, that cover MY34 to MY37.
Visible reflectance features of water ice include an average high reflectance, relatively constant over the VIS range, as well as asymmetric overtone absorption bands at 800nm, 840nm, and 1030nm. In CaSSIS colors, the strongest signature of water ice is the reduction of the strong visible red slope of the dust. It has been demonstrated experimentally that CaSSIS color criteria can help differentiate between different types of ice and dust mixings [7].
Observational results :
In accordance with previous studies, [5,8], Louth ice mound appears generally homogeneous, with little to no significant spatial variations in its global albedo and colour. This is not the case in two particular observations, taken on 15.02.2022, and on 28.03.2022, respectively. A dark pattern appeared and displayed changes in the eastern direction (in Fig.1, the red circle shows the area of change).
Fig.1 : Two CaSSIS images (MY36_018836_078 and MY36_018951_106) of Louth crater,
taken in early martian autumn. The two images are NIR/PAN/BLU composites.
We searched for reports of similar events in the literature, and only found one study [9] reporting an ‘unexplained dark patterning evolution’ in MRO - CTX images. We found other occurrences of dark patterns in MRO - HiRISE [10], in 2010, 2022, and 2023. Fig. 2 displays two examples of observations, both with HiRISE and CTX, in 2022 and 2023.
Fig.2: Two occurrences of dark patterns in 2022 (A) and 2023 (B)
The CTX observation shown in Fig.2 A was taken 14 days before the CaSSIS image MY36_018836_078 shown in Fig 1. A notable eastward progression can be distinguished.Each occurrence is different in shape, shows strong seasonality (appears at the end of summer, evolves through the autumn equinox), as well as rapid evolution (~tens of Martian days). The dark patterns disappear during each wintertime, showing a white mound each following spring. This indicates that the mechanism that produces these patterns is related to seasonal effects.
Preliminary spectral measurements of the ice in CaSSIS images show that the signal is largely dominated by the dust signature. We hypothesize that the dark marks could be caused by either deposition from the adjacent dune field, or local sublimation events.
What’s next ?
While continuing on spectral analysis with CaSSIS, we would like to explore the NOMAD [11] dataset to search if outliers in atmospheric water vapor content are correlated with the dark patterns occurrences. A ‘cloud’ can be seen in the MY36_018951_106 observation displayed in Fig.1. We do not know if this is linked to the formation of the North Polar Hood, or to the event itself. Thirdly, we will make use of the Mars Climate Database [12] to explore the temperature and relative humidity fields around Louth. Lastly, we will perform a similar study on Korolev crater. It is less clear than in Louth, but some structures appearing in early autumn resemble the ones that have been described here.
Acknowledgments:
This work has been developed in the framework of the National Center for Competence in Research PlanetS funded by the Swiss National Science Foundation (SNSF).CaSSIS is a project of the University of Bern and funded through the Swiss Space Office via ESA's PRODEX programme. The instrument hardware development was also supported by the Italian Space Agency (ASI) (ASI-INAF agreement no. 2020-17-HH.0), INAF/Astronomical Observatory of Padova, and the Space Research Center (CBK) in Warsaw.
References:
[1] Leighton, et al.. Sci. Vol.153, (1966).
[2] Brown, A J. et al. Icar., Vol.196, (2008).
[3] Armstrong, J.C, et al. Icar. Vol. 174 (2005).
[4] Conway, S. et al. Icar. Vol .220. (2012).
[5] Bapst, J. et al. Icar., Vol. 308,(2018)
[6] Thomas N et al. Space Sci. Rev. Vol. 212 (2017)
[7] Yoldi,Z. et al,Icar,Vol.358, (2021)
[8] Bapst J , Byrne S LPSC, Abstract #6097 (2015)
[9] Brown AJ et al. arXiv:1711.06372 (2017)
[10] McEwen,A.S., et al. Journal of Geo. Research: Planets 112.E5 (2007).
[11] Vandaele, AC, et al. Pl. and Space Sci. 119 (2015)
[12] Millour, E, et al. , EPSC2015-438, Vol. 10 (2015)
How to cite: Azevedo (1), M., Pommerol (1), A., and Thomas (1), N.: Exploring the seasonal variability at Louth crater ice mound with orbital colour imaging , Europlanet Science Congress 2024, Berlin, Germany, 8–13 Sep 2024, EPSC2024-611, https://doi.org/10.5194/epsc2024-611, 2024.
The 2-decade long observation time series of the High Resolution Stereo Camera (HRSC) [1] onboard Mars Express provides the precious opportunity to search for long-term surface changes on Mars in comparison to other multi-temporal image data of even earlier missions. Previous works on the topic of Martian change detection have mostly focused on either different sensor types (e.g., CaSSIS data [2] and MCC data [3]) or on short term and small-scale processes (e.g., gully formation [4] and impact cratering [5]). Surface changes on Mars that are not related to seasonal processes or are often too small-scale to be observed with medium- or low-resolution images occur very slowly. Besides impact cratering, aeolian erosion and deposition are the most important geological processes that cause perceptible surface changes today. As the winds on Mars have a low transport capacity due to the thin atmosphere, slow large-scale changes can best be observed in images with long time intervals. The identification of such long-term surface changes will help gaining a better understanding of Mars’ surface-atmosphere interactions and its general surface evolution.
This work starts out by visually comparing Viking orbiter images (from 1975 to 1980) and HRSC images (from 2004 to today) to find changes. We will additionally use image data from other sensors, such as CTX and MOC, to include observation with different spatial resolutions and to narrow down the time of the possible change process. The changes to be expected are wind streak, sand sheet and dust deposition (albedo) changes as well as long-term changes of the polar caps, slope-streak development and larger dune migration. As a start, this work focusses on wind streak changes, which are easy to detect and confirm. We found a significant amount of modified wind streaks around impact craters with changing orientation (azimuth) and/or changes in size. We will present first results from dark and bright (Figure 01) wind streaks in various regions on Mars, which indicate both depositional and erosional wind activity. Additionally, we will use the iron oxide ratio, a remote sensing index that uses the ratio of the red and blue wavelengths to visualize oxidized material on the surface [6], to visualize changes in depositional activity between HRSC images from different acquisition times. In a later step, we try to link the observed changes to specific atmospheric events that caused them (e.g., global dust storms). An overarching objective of our work is to assess the usability of HRSC data for detecting changes on the Martian surface and to provide a dataset of multi-temporal images that can be used for future studies and analysis of specific areas or features of interest.
Figure 01: Comparison of bright wind streaks in Syrtis Major, where we already found a significant amount of wind streaks with changing azimuth and/or changing size. Image A shows the area in the Viking MDIM2.1 Grayscale Global Mosaic with a resolution of 232 m. Image B shows the area in the HRSC image hm612_0000 with a resolution of 200 m. Sub-image a and b show the corresponding detailed section for a better analysis of the changing azimuth and/or size of the three wind streaks.
References
[1] Jaumann, R., et al., (2007). The high-resolution stereo camera (HRSC) experiment on Mars Express: Instrument aspects and experiment conduct from interplanetary cruise through the nominal mission. Planetary and Space Science 55, 928-952.
[2] Rangarajan, V., Tornabene, L., Osinski, G., Conway, S., Seelos, F., Silvestro, S., . . . Cremonese, G. (2023, January 26). Change detection and monitoring of active Martian surface phenomena. Icarus.
[3] Misra, I., Rohil, M., Moorthi, S., & Dhar, D. (2021). Mars Surface Multi-decadal Change Detection Using IRSO's Mars Color Camera (MCC) and Viking Orbiter Images. Springer Nature Singapore Pte Ltd.
[4] Pasquon, K., Gargani, J., Massé, M., & Conway, S. (2016, April 4). Present-day formation and seasonal evolution of linear dune gullies on Mars. Icarus.
[5] May, S. (2018). METEOR IMPACT DETECTION ON MARS WITH CHANGE DETECTION FRAMEWORK.
[6] Segal, D. "Theoretical Basis for Differentiation of Ferric-Iron Bearing Minerals, Using Landsat MSS Data." Proceedings of Symposium for Remote Sensing of Environment, 2nd Thematic Conference on Remote Sensing for Exploratory Geology, TX (1982): pp. 949-951." (ESRI, 2018)
How to cite: Schöpf, A., Tirsch, D., Jaumann, R., and Matz, K.-D.: Long Term Change Detection on Mars using HRSC images in comparison to other multi-temporal image data sets, Europlanet Science Congress 2024, Berlin, Germany, 8–13 Sep 2024, EPSC2024-201, https://doi.org/10.5194/epsc2024-201, 2024.
We are deriving insights about the climate evolution of Mars by automating surface mapping and monitoring with AI models. We are also analysing these models’ uncertainty and decision-making process with explainable AI and uncertainty estimation techniques. We focus on ice block fall detection at the north polar region and polygon detection globally.
The north polar ice cap on Mars is comprised of ice layers relating to the climate cycles of Mars and this way it preserves a ∼ 4 million years climate change record of the planet. These layers are exposed at the marginal steep scarps of the cap, that are currently active with avalanches and block falls. We are monitoring the mass wasting activity at the north polar region by detecting ice blocks [1] and their sources [2]. We estimate the current erosion rates of all scarps resulting in a detailed map of how this extensive ice-layered dome is being shaped today.
At the mid-latitude regions, where large volumes of excess ice exist, young patterned ground resembles glacial and periglacial patterns on Earth. Can freeze-thaw cycles have recently thawed the permafrost on Mars to produce these landforms? This would have implications for the recent hydrologic past of the planet. We detect young ice-wedge polygons to determine their distribution and relationship to the topography with the potential of elucidating the formation mechanism and the role of liquid water in the recent past of Mars.
We use AI models to automate surface mapping and monitoring, because they outperform all other methods. However, they are treated as black box systems. We want to know why a model produces a specific response and how certain it is about the correctness of each result. To answer these questions, we put together an application-independent framework that deploys uncertainty estimation and explainable AI methods to provide insights into the decision-making process and assess the uncertainty of the results [3], in this project the uncertainty of the surface mapping.
References:
[1] Martynchuk O. et al., Computer vision model for monitoring mas wasting activity in the Martian North Polar region. EPSC 2024.
[2] Su S. et al., Current mass wasting on icy scarps of Mars: A comprehensive mapping of ice-fragments in the north polar area. AGU 2023.
[3] Fanara L. et al., W2: How Certain and Why? Uncertainty Estimation and Explainable AI Applied on Mars Projects. AGU 2023.
How to cite: Fanara, L., Su, S., Martynchuk, O., Hauber, E., and Gwinner, K.: AI-driven surface mapping and explainable AI for climate insights on Mars, Europlanet Science Congress 2024, Berlin, Germany, 8–13 Sep 2024, EPSC2024-948, https://doi.org/10.5194/epsc2024-948, 2024.
Previous research has suggested that large floods in the Elysium mons region, Mars, may have melted significant amounts of ice in the late Amazonian epoch. Recent work has proposed that there may be remnants of ice-rich layers in the subsurface of the Medusa Fossae Formation (Watters et al., 2024), close to Elysium mons, see Figure 1. In this work we investigate the plausible presence of ices in Athabasca Valles region, which is located at the southern flank of Elysium Mons and close to the Medusa Fossae Formation (MFF).
Figure 1. Situation map of the area of interest considered in this study.
Athabasca Valles consists of a group of subparallel valleys with NE-SW orientation and SW vergence whose source area is located at 10ºN, 157ºE. This area has a consistent slope that stops at Cerberus Palus, see Figure 1. There are currently two different models to explain the recent geological history of Athabasca Valles: one of them postulates the existence of considerable amounts of ice in the subsurface that interacted with magma (Cassanelli and Head, 2018), whereas the other one considers only volcanic activity (Ryan and Christensen, 2012; Miller et al., 2023).
We have investigated the Athabasca Valles region to evaluate the possible presence of ice in the recent past. Using MOLA, THEMIS, HRSC, CTX and HiRISE observations, we find grooved surfaces at the bottom of the valleys, hills with nunatak like shapes, potential drop stones, surface features like polygonal patterned floors and pingo-like landforms, see Figure 2, as well as some other periglacial related landforms (Balme and Gallagher, 2009; Yakovlev et al., 2021). All of these features are caused by the sustained presence of ice.
Figure 2. CTX and HiRISE view. Top left: Eroded impact crater surrounded by grooved surfaces and possible dropstones, in the MurrayLab_CTX_V01_E152_N08 tile. Top right: Pingo like features and polygonal patterned floors in the HiRISE HI_046690_1900_063437_1900 image. Bottom left: Possible moraines and grooved surfaces observed in MurrayLab_CTX_V01_E156_N08 tile. Bottom right: nunatak-type mount observed in MurrayLab_CTX_V01_E156_N08 tile. All images have the same orientation.
We also observe the existence of a platy region with fractures that may be attributed to lava-ice interaction, see Figure 2. Using crater counting chronology methods, we have dated the platy surface structures and estimated an age of 29 Ma. We propose that the existence of ices under the surface 29 Ma ago caused the movement of the platy surface structures downstream of Athabasca Valles because of the lava-ice interaction. The valley has a consistent slope that stops at Cerberus Palus, and it is reasonable to think that ice or melted ice may have moved along this slope accumulating at Cerberus Palus.
Figure 3. THEMIS Thermal image of a fractured plate structure, where the plates show low thermal inertia whereas the inter-plate regions show high thermal inertia. These two differentiated parts have been dated using crater counting methods (see inset).
Using SHARAD data, we find bright radar reflectors in the accumulation area of Cerberus Palus, which we interpret as potential preserved subsurface layers of ice pore-filling materials, remains of previously existing ices, see Figure 4. We have modelled the temperatures of an hypothetical ice sheet under the surface of this region using the same methodology described by Egea et al. 2022 (Egea-González et al., 2022) for the North Pole region. Our analysis shows that subsurface ice can be thermally stable under the present-day thermal environment.
Our observations are consistent with the possible interaction of lava and ices in Athabasca Valles around 29 Ma ago, and the present-day preservation of ice in the subsurface at the end of the valley, in Cerberus Palus.
Figure 4. Example of one radargram where we can observe the existence of subsurface reflectors at the end of the basen.
Acknowledgements
M.-P.Z. and F. M. were supported by grant PID2022-140180OB-C21 funded by MCIN /AEI /10.13039/501100011033 / FEDER, UE. The work of F. Mansilla is supported by the grant PRE2020-09170, founded by MCIN/AEI/10.13039/501100011033.
References
Balme, M. R. and Gallagher, C.: An equatorial periglacial landscape on Mars, Earth Planet. Sci. Lett., 285, 1–15, https://doi.org/10.1016/j.epsl.2009.05.031, 2009.
Cassanelli, J. P. and Head, J. W.: Large-scale lava-ice interactions on Mars: Investigating its role during Late Amazonian Central Elysium Planitia volcanism and the formation of Athabasca Valles, Planet. Space Sci., 158, 96–109, https://doi.org/10.1016/j.pss.2018.04.024, 2018.
Egea-González, I., Lois, P. C., Jiménez-Díaz, A., Bramson, A. M., Sori, M. M., Tendero-Ventanas, J.-Á., and Ruiz, J.: The stability of a liquid-water body below the south polar cap of Mars, Icarus, 383, 115073, https://doi.org/10.1016/j.icarus.2022.115073, 2022.
Miller, R. C., Grima, C., Gulick, S. P. S., Goudge, T. A., Russell, A. T., Perry, M. R., Putzig, N. E., and Campbell, B. A.: Dynamic development of the Athabasca Valles outflow system from volcanic facies and 15 m scale roughness, Icarus, 115691, https://doi.org/10.1016/j.icarus.2023.115691, 2023.
Ryan, A. J. and Christensen, P. R.: Coils and Polygonal Crust in the Athabasca Valles Region, Mars, as Evidence for a Volcanic History, Science, 336, 449–452, https://doi.org/10.1126/science.1219437, 2012.
Watters, T. R., Campbell, B. A., Leuschen, C. J., Morgan, G. A., Cicchetti, A., Orosei, R., and Plaut, J. J.: Evidence of Ice‐Rich Layered Deposits in the Medusae Fossae Formation of Mars, Geophys. Res. Lett., 51, e2023GL105490, https://doi.org/10.1029/2023GL105490, 2024.
Yakovlev, V., Horelik, S., and Lytvynenko, Y.: On the cryogenic nature of the large hills of Mars, Planet. Space Sci., 208, 105340, https://doi.org/10.1016/j.pss.2021.105340, 2021.
How to cite: Mansilla Nuñez, F., Zorzano, M.-P., and Ruiz, J.: Potential existence and preservation of subsurface ice in the Athabasca Valles region, Mars., Europlanet Science Congress 2024, Berlin, Germany, 8–13 Sep 2024, EPSC2024-361, https://doi.org/10.5194/epsc2024-361, 2024.
Introduction
Orbital and in situ images have revealed past surface water activity on Mars. Morphological observations include valley networks [1, 2] and the presence of a few hundred paleolakes [3-5]. Jezero crater, which is the landing site for the Perseverance rover, is one of the most extensively studied palaeolakes today. This site is of interest for understanding ancient martian hydrologic activity, and for finding potential traces of past life. Current observations suggest that Jezero crater was fed by two inlet valleys [6] which are at the origin of two sedimentary fan deposits, interpreted as deltaic deposits [6, 7]. An outlet valley, Pliva Vallis, is present on the eastern crater rim [6] raising the question of whether the lake system operated mainly as an open basin, or as a closed basin system with episodic overflows. Resolving this uncertainty would improve our understanding of the Pliva Vallis’ evolution within time and consequently our knowledge of the history of Jezero’s lake.
Observations and interpretation
We performed a qualitative morphological study of the outlet valley using digital elevation models and orbital images (CTX and HiRISE data) acquired by Mars Reconnaissance Orbiter. First, we observed stratified and re-incised sedimentary deposits on the floor and inner walls of Pliva Vallis that we infer are a result of the fluvial activity of this valley. Alternating fine deposits and strata containing meter-scale boulders reflect the variation of flow intensity over time. Regarding the morphometry of the outlet valley, we observed several topographical features that are atypical for overland flows [8].
Indeed, the decrease of the valley width and depth from upstream to downstream, the presence of a perched channel and the observations of several topographic rises along the valley floor suggest a valley with a discontinuous activity and at least two episodes of energetic overflow. In addition, a few meters from the start of Pliva Vallis, we noticed the presence of four mains topographical steps within the wall of the valley, which are marked by the four colored lines in Figure 1 and are interpreted as four bedrock incisions terraces. This morphological observation confirms the episodic activity of Pliva Vallis with four rapid flow events caused by four phases of breach in the eastern rim of Jezero crater.
These observations suggest a scenario of lake overflow with at least four different events, responsible for the progressive and discontinuous incision of the outlet valley. Thus, we propose that the Jezero’s crater lake overflowed sporadically during the four breach events before emptying completely as a closed system though evaporation and infiltration, with limited fluvial input [9].
Modeling of the breach erosion processes
To give a first estimate of the duration of these four episodes of lake overflow and valley incision, we use a simple 0-D mathematical model, simulating valley formation by breach erosion and crater lake overspilling [10, 11]. This model provides a simple simulation of breach formation, particle transport and erosion rate in the outlet valley during time. Considering the imposed assumptions, (e.g., uniform valley geometry, no pre-existing valley, no downstream change in flow velocity, etc.), we applied the model to the Jezero’s crater lake in order to simulate the four overflow episodes previously identified, using the valley width and depth and the local slope measured for each episode where the four erosional terraces are identified. We assume a flow into the crater of about 100 m3/s, and we found that varying this value has little effect on our results given the order of magnitudes differences in discharge rate between this steady-state process and the overflows. Figure 2A shows the decrease of particle transport (D50 of 0.076 m [12]) over time for each event and for a range of slopes. As soon as the breach occurs, the flow loses energy progressively at first and then rapidly, until it can carry no more particles. The erosion rate can also be calculated during lake emptying for each episode (Figure 2B). Over time, erosion slows and tends to become zero as the flow decreases and loses energy. Modeling results suggest that each flooding and incision event in the outlet valley lasted less than a few weeks, or even a few days for some episodes. The time scales calculated with this model are consistent with our interpretation that flow events in the outlet valley were rapid and discontinuous.
Conclusion and outlook
The study of the outlet valley of Jezero crater helps us to understand better the activity of this lake. Our observations allow us to propose a new scenario of evolution of the Jezero’s crater lake - a closed basin system which was episodically and temporary opened during at least four identified overflow events.
References
[1] Pieri, D. C. (1980) Science, 90, 895–897.
[2] Carr, M. H. (1987) Nature, 326, 30–35.
[3] De Hon, R. A. (1992), Earth, Moon and Planets, 56, 95–122.
[4] Cabrol, N. A. and Grin, E. A. (1999), Icarus, 142, 160–172.
[5] Wharton, R. A., et al. (1995), Journal of Paleolimnology, 13, 267–283.
[6] Fassett, C. I. and Head, J. W. (2005), GRL, 32, L14201.
[7] Schon, S. C. et al. (2012), PSS, 67, 28–45.
[8] Schumm, S. A. and Ethridge, F. G. (1994), SEPM Society for Sedimentary and Geology, book.
[9] Mangold, N. et al., (2024) Constraints on Jezero paleolake history from its fluvial input, Abstract for The Tenth International Conference on Mars.
[10] Warren, A. O. et al. (2021), EPSL, 554, 116671.
[11] Holo, S. J. and Kite. E. S. (2019), 50th LPSC, Abstract #2481.
[12] Mangold, N. et al. (2024), JGR Planets, 129, e2023JE008204.
How to cite: Villette, J., Mangold, N., Conway, S., Le Deit, L., and Kite, E.: The evolution of Pliva Vallis, the outlet valley of the Jezero's crater lake on Mars, Europlanet Science Congress 2024, Berlin, Germany, 8–13 Sep 2024, EPSC2024-236, https://doi.org/10.5194/epsc2024-236, 2024.
Introduction
Hebrus Valles (HV) and Hephaestus Fossae (HF) are well-preserved Early Amazonian outflow channel systems carved into bedrock in SE Utopia Planitia, Mars (17-25 °N, 118-129 °E, Fig. 1). They exhibit a diverse set of morphologies indicative of formation by one or more liquid water outflow events (Christiansen, 1987; http://ntrs.nasa.gov/search.jsp?R=19870019962). However, little is known about their history, including both the origin and fate of the water and resulting sediments. This represents a significant gap in our understanding of geologic processes involving liquid water occurring in the Amazonian Period.
Thanks to extensive coverage by recent datasets, it is now feasible to study the evolution of the HV-HF outflow channel systems with an integrated analysis of diverse and complementary data, including high-resolution visible and infrared imagery mosaics. We performed chronostratigraphic geologic mapping of the HV-HF region followed by a detailed reconstruction of the sequence of geological processes and events based on the identification of morphological and thermophysical facies. Then, we employed impact crater statistical analysis to determine geologic unit and outflow channel ages.
Geologic mapping
The oldest unit in the study area is the Nepenthes flow unit, a complex layered volcanic and sedimentary unit that was previously identified in the adjacent Nepenthes Planum region (Skinner and Tanaka, 2018; doi:10.3133/sim3389). On top of it lies the Utopia lower unit, which also corresponds to a sedimentary unit identified older geologic maps (Tanaka, 2005; https://pubs.usgs.gov/sim/2005/2888/; Skinner and Tanaka, 2018; doi:10.3133/sim3389). Moving up in the stratigraphic column, we found three additional units: the Utopia lowland unit, the Utopia lumpy unit, and the Utopia lobate unit. Based on morphological facies analyses and the observation of clear and distinct stratigraphic contacts, we determined that the Utopia lumpy unit and the Utopia lobate unit originated from subsequent and distinct tsunami-like events (e.g., Rodriguez et al., 2022; doi:10.1038/s41598-022-18082-2) transporting sedimentary materials across Utopia Planitia. Instead, the Utopia lowland unit and the Utopia lumpy unit are not delimited by clear stratigraphic boundaries, suggesting that they may be different facies of the same chronostratigraphic unit. Three younger units overlie the Utopia units. In the NE corner of the geologic map lies the Elysium volcanic unit, which consists of volcanic flows related to Elysium Mons volcanic activity. To the south, we identified two additional volcanic and tectonic units: the Elysium platy unit and the Elysium chaos unit.
Figure 1: Chronostratigraphic map of the HV-HF region.
Age measurements
We conducted impact crater size-frequency distribution (CSFD) analyses on each chronostratigraphic unit by recording the locations and diameters of approximately 10000 impacts. We find formation ages for the Utopia units ranging from 2.9 Ga to 3.4 Ga (Fig. 2), placing the ages of units carved by channels between the Late Hesperian and Early Amazonian epochs. We also found evidence of resurfacing events occurring across three of the Utopia units at 2.2-2.3 Ga and a younger resurfacing event occurring at 1.7 Ga (Fig. 2). We interpret these events to be a combination of erosion of the Utopia Planitia plain-forming terrains and possible accumulation of volcanic sediments mantling the eastern half of the study area, perhaps associated with a peak in volcanic activity around 2-2.5 Ga (Platz and Michael, 2011; doi:10.1016/j.epsl.2011.10.001). Stratigraphy tells us that the Hephaestus Fossae and Hebrus Valles channel systems are necessarily younger than the surfaces they cut through, indicating that the channels must be younger than ~2.9 Ga. CSFDs within HV and in its distributary region yield ages of 1.8 Ga and 1.9 Ga, respectively (Fig. 3). Similarly, buffered crater counting of HV and HF yields ages of 2.1 Ga and 1.8 Ga, respectively (Fig. 3). These results confirm that the channels formed in the second half of the Early Amazonian about 1.5 Gy after the formation of the Utopia units, meaning that surficial aqueous activity in Utopia Planitia was occurring well into the Amazonian period.
Figure 2: CSFD analyses for the Utopia units with model formation ages (red) and resurfacing events (blue).
Figure 3: CSFD analyses for the HV and HF channels showing their model formation ages.
Origin and evolution of the outflow channels
The HV and HF channels originate from two deep pits within the Utopia lower unit (Fig. 1). We identified several elongated ridges protruding through the floor of these pits, which we interpret as remnants of magmatic intrusions that cracked the cryospheric seal of an ancient pressurized aquifer, as previously proposed for morphologically similar features in Cerberus Fossae (Head et al., 2003; doi:10.1029/2003GL017135). The morphology of the pits and the proximal sections of the channels are entirely compatible with morphologies observed in flume experiments where subsurface water discharge from a pressurized aquifer cause erosion and formation of terraces and deep source depressions within poorly consolidated sediments (Marra et al., 2014; doi:10.1002/2014JE004701). We reconstructed the initial phases of pit formation and channel incision in Fig. 4.
The pressurization of the aquifer can be explained by two mechanisms: heating of the aquifer by a magmatic intrusion that then cracked the cryospheric seal (Delaney, 1982; doi:10.1029/JB087iB09p07739) and/or the presence of an hydraulic gradient in an extensive cryospherically-sealed aquifer (e.g., Palumbo and Head, 2020; doi:10.1029/2020GL087230.). We find that both mechanisms could have contributed to the water discharge, and that the magnitude of their individual contributions depended on the permeability of the aquifer, because low permeability is required to maintain pressurization in the case of magmatic heating. We also note that the magmatic driver of pressurization and cryospheric cracking implies that the water release must have been modulated by magmatic activity, and thus we find it likely that multiple long-lived water discharge events occurred in this region until magmatic activity waned.
Figure 4: Step by step diagram of initial pit and channel formation. (left) A cryospheric seal confines a deep aquifer. Polygonal fractures are already present on the surface. (center) Magmatic intrusions crack the cryospheric seal leading to ponding of water, the formation of pits, and initial downslope flow on the surface. (right) Continuing water release deepens the source pits and carves channels in HV and HF, often following pre-existing fractures.
How to cite: Nerozzi, S., Spurling, R., and Holt, J.: The Fluvial and Volcanic History of Hebrus Valles and Hephaestus Fossae on Mars, Europlanet Science Congress 2024, Berlin, Germany, 8–13 Sep 2024, EPSC2024-648, https://doi.org/10.5194/epsc2024-648, 2024.
1.INTRODUCTION
Here we simulate the complete process of channel carving and the development of a valley-fan type system in the Coprates Catena region on Mars (Figure 1). Previous works [1,2,3] hypothetize that this stepped fan-like structure was formed in different stages, with an intermediate time-lapse. We reproduce the initial and final phases to analyze their different conditions and determine whether they represent alluvial fans (subaerial) or deltas (subaqueous). By iteratively combining topographic reconstructions and mechanical modeling, we can constrain the formation conditions of these surface processes that took place in the past on Mars’s surface, including if they are compatible with a permanent sheet of water covering the canyon (Figure 2).
Figure 1. Top left, location of Coprates Catena on the Mars globe (14º 59' S; 60º15' W), south of Valles Marineris. Below is a delimitation of the two episodes considered on a mosaic of CTX images, the subaqueous (oldest) in blue and the subaerial (youngest) in orange.
2.METHODOLOGY
We begin with a "backward reconstruction,” modifying the topography to approach the area shape before the triggering event [4,5]. We subtract the volume of fan-shaped material, backfilling the channel with the equivalent mass (Figure 2). Through interpretive geology, we identify which points of the observed topography were not modified after the event and reconstruct the altered areas using a thin plate spline interpolation algorithm (Figure 3). The mobilized material, an initial condition necessary to feed the numerical model, is then obtained with the difference between both reconstructed topographies, which is then interpolated in an unstructured computational mesh.
Figure 2. Topographic profiles (see Figure 1) for the thalweg (blue) and slope edge for both the older (red) and younger (orange) episodes. The vertical black line marks the boundary between deposition and transport. The dashed blue line shows the paleolake level.
Figure 3. Above, points used for topographic reconstruction of the channel (Orange: youngest episode; Black: oldest episode; Blue: thalweg; Yellow: reference points outside the channel; White: points belonging to altered zones to be calculated). Below is a reconstruction of the channel and canyon using thin plate spline interpolation in MATLAB.
For the "forward reconstruction” of the event, a systematic numerical study is performed using a two-layer model based on the depth-integrated Navier-Stokes equations [6] with different constitutive models combined with bottom friction laws [7,8,9]. The resulting model allows the dynamic simulation of fluid displacement under subaerial and subaqueous conditions, providing the flow time and runoff distance, as well as the final volume and shape of the deposits.
Considering that this fan structure formed in several phases, with a time span in between [1,2,3], we have divided the event into two distinct episodes: The older episode (Figure 2, in blue), which is assumed to be a subaqueous deposition due to the presence of a deep lake within the canyon [2]; and the more recent episode (Figure 2, in orange), which is considered to be an alluvial fan that was deposited on top of the previous subaqueous delta [3].
3.RESULTS
Tectonics related to the Coprates horst/graben system were reactivated after the channel’s creation in the oldest episode (Figures 4E,4F), producing several contractions and faults that have altered its shape. Consequently, applying the mechanical model directly to the observed topography today results in the material not flowing properly. Therefore, a "backward reconstruction" is required to reverse these modifications without excessively altering the original shape of the surface so we can provide a valid input for the mechanical modeling. Thanks to the method used (Figure 3), we obtained the channel topographies preceding (Figures 4A,4E) and following (Figures 4B,4F) the two episodes, removing the sedimentary deposits at its mouth.
Figure 4. "Forward reconstruction" of the youngest (A-D, orange in Figures 1 and 2) and oldest (E-H, blue and red respectively in Figures 1 and 2) episodes. Topography before (A and E), after (B and F), and the event and initial condition were obtained with the difference between both (C and G). The result of the numerical modeling and comparison of the observed fan shape (bottom) and its reconstruction (top) are shown for the youngest (D) and the oldest (H) episodes.
For the "forward reconstruction,” we conducted multiple simulations of the formation of these deposits using different rheological laws for Newtonian, frictional, and Bingham fluids [7,8]. The mixture’s density, viscosity, and yield strength are related by the constitutive equation. The best match between simulations and actual observations is achieved for a Bingham-type mudflow consisting of a kaolinite-water mixture [4]. The interstitial water content is calculated from the empirical expression for kaolinite-type water-clay mixtures [10]. Table 1 shows the final parameters that best fit the observed morphologies.
Youngest episode | Oldest episode | |
Density (kg/m3) | 1722 | 1606 |
Viscosity (Pa·s) | 0.114 | 0.958 |
Elastic limit (MPa) | 1.53 | 0.07 |
Water content (%) | 55 | 62 |
Table 1. The final parameters used in the mechanical model to obtain the results are shown in Figure 4D (youngest episode) and 4H (oldest episode).
Regarding the most recent episode, our results (Figure 4D, Table 1) are compatible with a kaolinite-type clay-water mixture mudflow with an interstitial water content of about 55%. For the older episode, using the same material and water content as in this episode, the "forward" reconstruction of the event by numerical simulation does not reproduce the observed final shape and height of the deposit. With these same parameters and considering different depaleolakes depths filling the canyon, as suggested by [3], it does not match the observations either. However, by increasing the interstitial water content of the sliding mass to 62% and defining a 1500m deep water sheet, the reconstruction of the event does match the observations (Figure 4H, Table 1). This finding supports the hypothesis of two different episodes for the formation of the fan, with different amounts of water, and the existence of a paleolake of considerable depth during the oldest episode studied.
4.ACKNOWLEDGMENTS
A.Molina thanks EDRIM project (VAPC 202250I104), D.Pascual JAE Intro 2022 program (JAEINT_22_00950, JAEINT22_EX_0770), T.Martínez-Pérez PTA2022-021846-1(MCIN/AEl 10.13039/501100011033yFSE), and A.Caramanico INTIME project (Grant agreement ID: 823934; DOI 10.3030/823934).
5.REFERENCES
How to cite: Molina, A., Herreros, I., de Pablo, M. Á., Pascual, D., Caramanico, A., Martínez-Pérez, T., Gómez, B., Reboredo-Prado, M., and Haukes, C.: Reconstruction of overlapped subaerial and subaqueous deposits in Coprates Catena, Mars, Europlanet Science Congress 2024, Berlin, Germany, 8–13 Sep 2024, EPSC2024-829, https://doi.org/10.5194/epsc2024-829, 2024.
Please decide on your access
Please use the buttons below to download the supplementary material or to visit the external website where the presentation is linked. Regarding the external link, please note that Copernicus Meetings cannot accept any liability for the content and the website you will visit.
Forward to presentation link
You are going to open an external link to the presentation as indicated by the authors. Copernicus Meetings cannot accept any liability for the content and the website you will visit.
We are sorry, but presentations are only available for users who registered for the conference. Thank you.
Abstract
We present results of experiments performed inside a low-pressure chamber designed to investigate whether the volume of mud changes when exposed to a Martian atmospheric pressure. Depending on the mud viscosity, we observe a volumetric increase of up to 30% at the pressure of ∼6 mbar. The reason is that the low pressure causes instability of the water within the mud, leading to profuse bubble formation that increases the volume of the mixture. This mechanism bears resemblance to the volumetric changes associated with the degassing of terrestrial lava or mud volcano eruptions caused by a rapid ascend along the conduit and associated pressure drop.
Introduction
Subtle mounds have been discovered in the source areas of Martian kilometer-sized flows and on top of summit areas of some domes (Fig. 1; [1-2]). These features have been suggested to be related to subsurface sediment mobilization, opening questions regarding their formation mechanisms. Previous studies hypothesized that they mark the position of feeder vents through which mud was brought to the surface [1-2]. Two theories have been proposed: (a) ascent of more viscous mud during the late stage of eruption and (b) expansion of mud within the conduit due to the instability of water under Martian conditions.
Fig. 1. Examples of Martian mounds and knobs.
Mud volcanoes on Earth release mud with a wide range of viscosities [3]; likewise similar variations are also expected in the mud extruded on other planetary surfaces. So far, experimental studies have been conducted only for low viscosity mud under low pressure conditions [4-5], and the behavior of more viscous muds under such conditions remains unclear. To overcome this knowledge gap, we completed analog experiments, to investigate the behavior of various viscous muds under martian pressure conditions.
Methods
We performed a set of experiments using the Mars Simulation Chamber at the Open University (UK), in which we inserted a two-part test bed that contained a reservoir which was able to accommodate 600 ml of mud and a ∼10 cm thick layer of frozen icy-sandy infill as the surrounding (Fig. 2). The temperature of this infill was around -20°C before experiment started. No active cooling of the icy-sandy infill was used, hence the infill slowly warmed up. All experiments were completed before the temperature of the icy-sandy infill reached the melting point of the water ice. The temperature of the mud when poured into the container was either 0.5-3°C (further refer as “cold”) or 13-22°C (“warm”).
Figure 2. Schematic illustration showing the experimental setup inside the Mars Simulation Chamber.
Three different viscosities were used (Fig. 3) and they are further refer as “low”, “medium” and “high.” The mud mixtures were prepared by mixing deionized water with 1% w/w of dissolved magnesium sulfate salts (MgSO4) and clay content varying depending on the required viscosity. Magnesium sulfate salt was added to the water to achieve Earth's average river salinity, which enables the suspension of submillimeter clay particles within low viscosity mixtures. During the experiment, the pressure was gradually reduced from 1 bar to 5-7 mbar to achieve the martian surficial pressure. Two different speeds of pressure drops were utilized, namely the pressure was reduced within a timeframe of minutes (rapid) or more than an hour (slow).
Figure 3. Viscosity curves of “low”, “medium” and “high” viscosity aqueous bentonite.
In order to quantify volumetric changes of the mud samples, we used semi-manual and automatized image analyses using the PIV (Particle Image Velocimetry; [6]) and photogrammetry methods.
Results and discussion
Results revealed a significant volume increase during the experiments with slow depressurization, high mud viscosity and low initial mud temperature (Fig. 4). The volumetric change occurs due to the formation of water vapor bubbles, which are temporarily trapped within the mud. This phenomenon occurs since the bubble buoyancy is insufficient to overcome the drag force within the viscous material. Hence, these bubbles remain trapped in the mud allowing their gradual growth up to centimeter-scale sizes. During their volume increase, they push the mud out from the container resulting in horizontal and vertical propagation of the mud over cm-scales. In those experiments where the mud bulge freezes due to the evaporative cooling, the internal structure is kept in (or beneath) the icy crust. Our experimental approach reveals that mud with identical characteristics features different morphologies depending if its extrusion occurs on Earth or Mars pressure conditions.
Figure 4. Results of volumetric change measurements.
As the surface gravity on Mars is nearly three times smaller than that on Earth, we performed numerical calculations to reveal to which depth the mud undergoes boiling. We reveal that the depth of boiling would be nearly three times larger on Mars, reaching meters for sufficiently warm muds. As a consequence, the boiling and associated volumetric changes observed during our small-scale experiments may apply to meter-scales for Mars natural conditions . This suggests that the observed mounds and knobs associated with putative martian sedimentary volcanoes might indeed be related to mud volumetric changes in response to surface exposure. We also show that mud flows on Mars, and elsewhere in the Solar System, could behave differently to those found on Earth since mud dynamics are affected by the formation of bubbles in response to the different atmospheric pressures.
Figure 5. Simplified concept of gas migration and mud inflation due to the pressure drop.
Results of these experiments also suggest that other types of liquid, that are unstable in the low pressure environment, might behave similarly if their viscosity is high enough to prevent the bubble escape. The results presented herein also have implications for cryovolcanic phenomena on icy moons (e.g., Enceladus or Europa) or dwarf planets like Ceres.
References: [1] Komatsu et al., 2016. Icarus, 268; [2] Brož et al., 2019, JGR: Planets, 124(3); [3] Mazzini and Etiope, 2017, Earth-Science Reviews, 168. [4] Brož et al., 2020a, Nature Geoscience, 13(6); [5] Brož et al., 2020b, EPSL, 545; [6] Thielicke and Stamhuis, 2014, Journal of Open Research Software, 2(1).
How to cite: Broz, P., Krýza, O., Patočka, V., Pěnkavová, V., Conway, S., Mazzini, A., Hauber, E., Sylvest, M., and Patel, M.: Volumetric Changes of Mud on Mars: Evidence from Laboratory Simulations, Europlanet Science Congress 2024, Berlin, Germany, 8–13 Sep 2024, EPSC2024-277, https://doi.org/10.5194/epsc2024-277, 2024.
Introduction: The Thumbprint Terrains (TT) are intriguing geological formations that can be observed in the northern plains (NP) of Mars. TT are commonly described as an alternation of parallel alignments of 10s of meters high high-albedo ridges and dome-like mounds, separated by shallow depressions with contrasting low albedo[1]. These smooth surface deposits are composed of fine-grained loose materials[2]. TT mounds have been tentatively proposed as being formed by: explosive volcanism[3], glacial processes[1,4], sedimentary volcanism[2,5,6], or tsunami-driven events formed after giant impacts[2,6,7]. TT formation may be recent (Late Hesperian-Early Amazonian), period overlapping with the outflow channel activity timing and a possible transient Hesperian Ocean on Mars[7]. However, our lack of knowledge about the nature of these deposits persists, not least because no mineralogical clue has been found[8]. This study proposes to provide constraints on the nature of these deposits and their possible subsurface layering, thanks to the recent observation of hydrated silica (HySi) and sulfates (PHS) on the TT of Acidalia Planitia[9].
HySi is systematically associated with TT’s mounds [Fig. 1A,B,C,D]. HySi is only observed in these mounds and seems to be directly linked to the activity of these. We calculated spectral criteria on HySi CRISM detection to decipher the type of silica and to infer its possible geological origins[10]. Silica possibly occurs as altered volcanic glasses, or as dehydrated opals. These criteria also suggest that the silica was formed by low-T fluid-rock interactions and/or late-stage water-limited alteration. We propose that HySi in the Acidalia’s TT cones represents weathered, Si-rich fine materials. Possibly in the form of low-density volcanic ashes, HySi was extruded to the surface by sedimentary volcanism involving low quantity of fluids/volatiles (no surface flow observed). Such type of mounds could be indicative of sand volcano-like mounds and/or hydrodynamic blowouts whose sources, constrained by the thickness of the TT (less than 100m). This low-lying origin is confirmed by the results obtained by calculating the estimated extraction depths of such HySi-rich sources by sedimentary volcanism using a buoyancy-driven model in subaerial environment[11]. HySi-rich materials seem to be sourced from several 10s of m underground (between -10 to -80 m), from subsurface layers that are internal to the TT.
PHS are mainly associated with impact craters with diameters less than or close to ~1 km, and mostly located in their (sometimes lobate) ejecta [Fig.1A;B]. As such small impacts can excavate materials up to 100-150 m depth, PHS presence in these may indicate the presence of sulfate-bearing lithologies in the TT’ subsurface or in the underlying units. This is inferred from observations such as the one presented in Fig.1B where two impacts excavate PHS in two different settings. Further evidence of the presence of sulfates within the TT (or in deeper underlying units) is the observation of PHS in one large 165 m high mound that also display HySi at its base [Fig. 1C]. Applying the same buoyancy-driven model to this PHS mound, it returns that the PHS sources can be several hundred meters deeper (-200 up to -600 m), possibly out of TT’ stratigraphy. However, it is also possible that the sulfates present here have an origin directly within the TT. Indeed, when sedimentary volcanism occurs, it is common to have remobilization and incorporation of the crossed layers by the injected fluids or materials. This hypothesis of co-presence of PHS and HySi in the TT could explain the observation made of such high-mounds (Fig.1C).
Implications for Subsurface Stratigraphy. The detection of hydrated minerals confirms the sedimentary volcanism hypothesis for the origin of TT mounds. HySi-rich mounds indicate that subsurface localized pockets of hydrated materials are present several 10s of m deep within the TT’ stratigraphy. The nature of surface materials suggests that the TT are partly composed of Si-rich volcanic ashes, that, mixed with moderate quantities of over-pressurized fluids, were able to migrate to the surface through (explosive?) sedimentary volcanism. Highest mounds, enriched with PHS, can have sources that lie several hundred meters below the TT. In such a configuration, these sulfates-rich sediments are older than the “mostly Amazonian” Si-rich lithologies making up the TT and may be an inherent part of the stratigraphy composing the underlying “mostly Hesperian” Vastitas Borealis Formation (VBF), possibly in the form of buried evaporites.
Ongoing Perspectives. This study is currently being extended to numerous sites where sedimentary volcanism has been proposed on the basis of geomorphological observations[12] and where hydrated minerals have been detected[9]. Applied across all the NP, our study will enable us to better characterize the aqueous materials in the Martian subsurface. It will also provide constraints on the nature of the sedimentary volcanism-related edifices in various regions, but also on the origins of the VBF, for which numerous studies suggest a potentially “not so ancient” aqueous origin[13].
Acknowledgments. We acknowledge the support from the Agence Nationale de la Recherche (ANR, France) under the contract ANR-20-CE46-0013 entitled “PaleoSilica”, the Centre National d’Études Spatiales (CNES, France), the Centre National de la Recherche Scientifique (CNRS, France), and the French government under the France 2030 investment plan, as part of the Initiative d'Excellence d'Aix-Marseille Université - A*MIDEX AMX-21-RID-O47.
References. [1]Lockwood et al. (1992) 23rdLPSC. [2]Di Pietro et al. (2021) Icarus. [3]Frey & Jarosewich (1982) JGR Solid Earth. [4]Souček et al. (2015) EPSL. [5]Salvatore & Christensen (2014) JGR Planets. [6]Costard et al. (2017) JGR Planets. [7]Costard et al. (2019) JGR Planets. [8]Oelher & Allen (2010) Icarus. [9]Carter et al. (2023) 54thLPSC. [10]Pineau et al. (2020) Icarus. [11]Hemmi & Miyamoto (2018) Geosciences. [12]Broz et al. (2023) Earth Surf. Dyn. [13]Kreslavsky & Head (2002) JGR Planets.
Figure 1. A. to D. PHS (green) and HySi (cyan) CRISM detections in four sites within the Acidalia’s TT over CTX background, North is up. For each, coordinates of CRISM cubes are provided. Scale bars indicate 2000m.
How to cite: Pineau, M., Carter, J., Lagain, A., Mangold, N., Le Deit, L., and Quantin-Nataf, C.: Mineralogical Evidence of Sedimentary Volcanism for the Formation of the Thumbprint Terrains in Acidalia Planitia, Mars., Europlanet Science Congress 2024, Berlin, Germany, 8–13 Sep 2024, EPSC2024-906, https://doi.org/10.5194/epsc2024-906, 2024.
On Earth, the characteristics of fluvial erosion (drainage direction, discharge, drainage area, and channel complexity) depends on climate (rain fall) and tectonic development (uplift rate, slope, and relief). Mars is a planet that experienced erosion driven by liquid water in its history, but its tectonics are vastly different from Earth’s. The formation of the Tharsis dome, a vast volcanic province represents a major magmato-tectonic upheaval for the planet. This event occurred during the early history of Mars, when it had an active water cycle, evidenced by the formation of fluvial valleys that have been preserved until present. The timing of the rise of the Tharsis province and the carving of valley network are debated (Phillips et al., 2001; Bouley et al., 2016). If they simultaneously occurred, it is likely we would see the tectonic change in Mars’ surface due to Tharsis affect the characteristics of fluvial erosion as listed above. Conversely, if both events are separate in time then the valley networks will have formed on a strictly Pre-Tharsis Mars, or a Post-Tharsis Mars. Nonetheless it is important to settle this debate in order to determine precisely the surface conditions prevailing during a period of time when the red planet could have seen the development of life.
This study is the first to model the influence of the growth of Tharsis on the formation of the Valley networks. We present results from numerical simulations performed using a Stream Power Law (SPL) algorithm on Mars during the emplacement of Tharsis to assess how the patterns of drainage are affected by the tectonic development of the planet, in an attempt to reproduce the observed distribution of valley networks and their geometry. We find that the tectonic change due to Tharsis measurably affects drainage systems across the planet and adjust the timing until we reach a close match between our modelled network and the observed one. As Tharsis emplaces and regions uplift and subside in response, their local slope and relief is altered, having the effect of dispersing networks, lowering discharge, drainage area and erosion rate in uplifting regions and converging networks, increasing discharge, coalescing drainage areas, and increasing erosion rate in subsiding regions. This causes a preservation of older networks in the uplifting regions and a replacement of networks in subsiding regions, making it appear that certain valley networks are older and others are younger (Fig. 1). This suggests that not only is Tharsis emplacement necessary to produce the relief needed to form the observed networks on Mars today, but that a prolonged (and likely intermittent) period of fluvial activity on Mars must have occurred concurrently with the emplacement of Tharsis. In conclusion, Mars offers the opportunity to understand how landscape may evolve under a wider range of tectonic regimes, compared to Earth.
Fig 1. A map of Mars with total topographic change hypothesised to have been caused by Tharsis (Matsumaya and Manga 2010), overlain with SPL model results divided into 15°x15° bins and coloured based on when in the model time the valley networks best match with observations when the two are compared using a Discrete Fourier Transform (DFT) signal comparison. Where the observational data best matches model data Pre-Tharsis, at 3.8 Ga model time is marked in black, Mid-Tharsis at 3.65 Ga in dark gray, and Post-Tharsis at 3.5 Ga in light gray. Crosses on the map denote valley networks dated by Hoke & Hynek (2009) with their respective ages.
References
Bouley, S., Baratoux, D., Matsuyama, I., Forget, F., Séjourné, A., Turbet, M., and Costard, F.: Late Tharsis formation and implications for early Mars, Nature, 531, 344–347, https://doi.org/10.1038/nature17171, 2016.
Hoke, M. R. T. and Hynek, B. M.: Roaming zones of precipitation on ancient Mars as recorded in valley networks, Journal of Geophysical Research: Planets, 114, https://doi.org/10.1029/2008JE003247, 2009.
Matsuyama, I. and Manga, M.: Mars without the equilibrium rotational figure, Tharsis, and the remnant rotational figure, Journal of Geophysical Research: Planets, 115, https://doi.org/10.1029/2010JE003686, 2010.
Phillips, R. J., Zuber, M. T., Solomon, S. C., Golombek, M. P., Jakosky, B. M., Banerdt, W. B., Smith, D. E., Williams, R. M. E., Hynek, B. M., Aharonson, O., and Hauck II, S. A.: Ancient Geodynamics and Global-Scale Hydrology on Mars, Science, 291, 2587–2591, https://doi.org/10.1126/science.1058701, 2001.
How to cite: Davies, H. S., Bouley, S., Baratoux, D., and Braun, J.: What role did Tharsis formation have on the geomorphological history of Mars, Europlanet Science Congress 2024, Berlin, Germany, 8–13 Sep 2024, EPSC2024-503, https://doi.org/10.5194/epsc2024-503, 2024.
Tyrrhena Terra, a region located in the cratered Noachian highlands between Hellas and Isidis Planitia on Mars, is distinguished by its extensive presence of hydrated minerals. As a key region for understanding the early Martian aqueous environment, various evidence for hydrated alteration has been reported by previous studies (Loizeau et al., 2012; Rogers, 2011; Carter et al., 2013; Bultel et al, 2015; Viviano et al., 2023). However, the effects of varying crater scales on mineral formation and distribution have not yet been well understood.
In this comprehensive investigation, we identified hydrated minerals across Tyrrhena Terra (2°N-22°S, 64°E-104°E), using near-infrared hyperspectral data from the Compact Reconnaissance Imaging Spectrometer for Mars (CRISM). Along with high-resolution imaging from the Context Camera (CTX) and the High-Resolution Imaging Science Experiment (HiRISE), we explored the relationship between impact crater size and degradation rate and the diversity of hydrated minerals. Based on crater diameter, the observations were systematically categorized into five groups: away from craters, <5 km, 5-10 km, 10-20 km, and >20 km. Additionally, craters larger than 20 km were subdivided based on degradation morphology into three types (Mangold et al., 2012): type I (highly degraded), type II, and type III (least degraded).
Our findings showed a widespread distribution of phyllosilicates, including Fe/Mg-smectites, chlorite, kaolinite, mica, and their mixtures, as well as other hydrated minerals such as prehnite, zeolite, carbonate, and hydrated silica (Figure 1). We observed a significant increase in mineral diversity with larger crater diameters, even when excluding minerals located on central peaks (Figure 2). Such trend indicates that the subsurface compositions vary significantly with depth, since the post-date impact generated hydrothermal deposit mostly found in the central peak of craters. By excluding minerals on central peaks, we reduced the influence from impact-driven hydrothermalism for hydrated minerals formation, thus maximized the role of impact events to be the excavation of subsurface minerals.
Figure 1. The distribution of hydrated minerals in Tyrrhena Terra.
Figure 2. The variation of the hydrated minerals with the diameter of impact crater.
Notably, for craters larger than 20 km, the mineral diversity also shows a strong correlation with the degradation rate of craters (Figure 3). Only Fe/Mg-smectites and chlorite were observed in highly degraded type I craters, likely dating back to the Noachian period, while prehnite, zeolite, carbonate, and hydrated silica were more common in less degraded type II and type III craters. Moreover, the context of Fe/Mg-smectites exposures in Tyrrhena Terra is particularly intriguing. Fe/Mg-smectites were prevalent not only in type I craters, but also in ancient sediments that are incised by valley networks, isolated from impacts, and with small amount of Al-smectite mixtures observed (Figure 4c); as well as in exposures of small impacts (less than 5 km in diameter) on ancient sediments (Figure 4b, CRISM image FRT00014005 and FRT00024086). In contrast, the interiors of large, well-preserved craters exhibit a complex diversity of minerals, including those formed under relatively high temperatures, such as prehnite and zeolite. It appears that minerals formation involves processes beyond only impact events. Such difference revealed clear evidence for active surface alteration in Noachian period, resulting in the transformation of hydrated minerals.
Figure 3. The variation of the hydrated minerals with the degradation rate of impact crater larger than 20 km.
Figure 4. Key examples for the context of Fe/Mg-smectites and Al-smectites (mixed with Fe/Mg-smectite) in Tyrrhena Terra. (a) CRISM spectral ratios (top and middle frame) compared to Fe/Mg-smectites and Al-smectites laboratory spectra from USGS and RELAB (bottom frame). (b) Fe/Mg-smectites exposed by small craters on ancient sediments. (c) Fe/Mg-smectites on ancient sediments and isolated from impacts (in magenta), as well as small exposures of Al-smectites mixed with Fe/Mg-smectites (in cyan, extracted from Carter et al., 2023). The RGB channels are Band 233, Band 78, and Band 13 respectively for all CRISM images.
Our findings highlight a regional source-to-sink geological process where minerals were transformed and redistributed. These minerals are excavated by local impacts, subsequently altered by surface weathering processes, and transported through fluvial activity to their present locations. This suggests that more than one period of hydrated alteration was active in early Martian history, likely predating the formation of widespread valley networks, with each period lasting long enough for hydrated minerals to be formed or altered. This research offers new insights into the early Martian aqueous history and its past environmental conditions.
References:
Bultel, B., Quantin-Nataf, C., Andréani, M., Clénet, H., & Lozac’h, L. (2015). Deep alteration between Hellas and Isidis basins. Icarus, 260, 141–160.
Carter, J., Poulet, F., Bibring, J.-P., Mangold, N., & Murchie, S. (2013). Hydrous minerals on Mars as seen by the CRISM and OMEGA imaging spectrometers: Updated global view. Journal of Geophysical Research: Planets, 118(4), 831–858.
Carter, J., Riu, L., Poulet, F., Bibring, J.-P., Langevin, Y., & Gondet, B. (2023). A Mars orbital catalog of aqueous alteration signatures (MOCAAS). Icarus, 389, 115164.
Loizeau, D., Carter, J., Bouley, S., Mangold, N., Poulet, F., Bibring, J.-P., Costard, F., Langevin, Y., Gondet, B., & Murchie, S. (2012). Characterization of hydrated silicate-bearing outcrops in Tyrrhena Terra, Mars: Implications to the alteration history of Mars. Icarus, 219(1), 476–497.
Mangold, N., Adeli, S., Conway, S., Ansan, V., & Langlais, B. (2012). A chronology of early Mars climatic evolution from impact crater degradation. Journal of Geophysical Research: Planets, 117(E4).
Rogers, A. D. (2011). Crustal compositions exposed by impact craters in the Tyrrhena Terra region of Mars: Considerations for Noachian environments. Earth and Planetary Science Letters, 301(1-2), 353–364
Viviano, C. E., Beck, A. W., Murchie, S. L., Dapremont, A. M., & Seelos, F. P. (2023). Heterogeneity of the Noachian crust of Mars using CRISM multispectral mapping data. Geophysical Research Letters, 50(5), e2022GL102711.
How to cite: Wu, Y., Mangold, N., Liu, Y., Carter, J., Wu, X., Pan, L., Huang, Q., and Zou, Y.: Comprehensive analysis of the alteration of Tyrrhena Terra: Implications for source-to-sink processes on Mars, Europlanet Science Congress 2024, Berlin, Germany, 8–13 Sep 2024, EPSC2024-364, https://doi.org/10.5194/epsc2024-364, 2024.
Sulfate minerals are an integral component of the martian surface and understanding the formation and alteration of these minerals provides clues about their geochemical environment. One sulfate phase in particular has been intriguing Mars scientists for over 15 years. An unusual spectral band at 2.236 µm was discovered in CRISM spectra of Mars at the plateau bordering Juventae Chasma [1] and in Aram Chaos [2]. This spectral band does not line up with any known minerals, but is observed for FeSO4OH, a new mineral formed by heating hydrated iron sulfates [3]. Crystal structure diagrams indicate that FeSO4OH has a structure similar to that of szomolnokite (FeSO4•H2O), but with OH replacing H2O [4]. Experiments heating szomolnokite, rozenite, and melanterite in the lab resulted in production of some FeSO4OH at 150 °C from rozenite and melanterite after 30 minutes and complete transformation at 200 °C after 30 minutes, while reaction of szomolnokite required longer heating at 200 °C and/or elevated temperatures for transformation to FeSO4OH. Additional lab experiments also demonstrate that oxygen is required for reaction of these hydrated ferrous sulfates to form FeSO4OH [4].
Application of FeSO4OH spectra to Mars has benefitted from improved processing techniques [5] and mapping algorithms [6] for CRISM images that has enabled characterization of smaller spot sizes with cleaner spectra. Exposures of this unusual ferric sulfate phase with spectral features near 2.23 µm at Aram Chaos closely resemble pure FeSO4OH formed in the lab, while the thinner units on the Juventae plateau are either mixed with other components or represent incompletely formed FeSO4OH phases. This Fe hydroxysulfate is currently associated with monohydrated sulfate (MHS) outcrops at Aram Chaos, although polyhydrated sulfate (PHS) outcrops are also present nearby. In contrast, only PHS outcrops are currently observed on the Juventae plateau.
CRISM spectra with a spectral band at 2.225-2.238 µm were observed at several locations at Aram Chaos. The spectra of these units also contain accompanying features at 1.48, 1.82, 2.19, and 2.37 µm (Fig. 1). Small variations in the ~2.23 µm band are attributed to changes in the Fe-Mg chemistry. Pure FeSO4OH has a band at 2.236 µm, while heated FeMg-MHS has a band at 2.226 µm. The FeSO4OH units at Aram Chaos are typically found adjacent to MHS outcrops (Fig. 2), including both Fe-rich MHS (similar to szomolnokite) with bands near 2.11-2.12 and 2.40 µm and kieserite (MgSO4•H20) with spectral bands near 2.14 and 2.41 µm. Spectra of szomolnokite and kieserite measured at colder, Mars-like temperatures have bands at ~2.11 and 2.14 µm [7], similar to these observations. The MHS spectral units more consistent with kieserite are darker than the szomolnokite-like MHS units and are covered by debris and ripples (Fig. 2C). Some of the MHS units at Aram Chaos may have formerly been mixtures of szomolnokite and kieserite, where the szomolnokite transformed to FeSO4OH and the kieserite remained. Alternatively, polyhydrated Fe and Mg sulfates may have been present that altered to form FeSO4OH, szomolnokite, and kieserite, depending on variations in the geochemistry. The reaction to form FeSO4OH proceeds as Fe2+ in szomolnokite is oxidized to Fe3+ while the H2O loses a proton to form OH. Some of the MHS outcrops include weak bands near 2.23 µm, indicating partial alteration to form a mixed phase containing some MHS and some FeSO4OH (Fig. 3). The PHS spectra contain bands near 1.44 and 1.93-1.95 µm and a drop in reflectance near 2.42 µm (Fig. 3), similar to spectra of rozenite (FeSO4•4H20) and starkeyite (MgSO4•4H20).
Spectra at the Juventae plateau were collected from thin light-toned layered deposits, including spectral signatures due to PHS and FeSO4OH, and pyroxene-bearing units (Figs. 3-4). The stratigraphy of the outcrops shows a pyroxene-bearing substrate below the light-toned layered materials and a different pyroxene-bearing caprock unit covering the Fe sulfates (Fig. 4). Thin units containing spectral features consistent with PHS (blue) and FeSO4OH (red) are observed, and some of these thin units include spectral features due to both materials. Morphologies of these primary four units are displayed in Fig. 5. The pyroxene bearing substrate (dark cyan) is flatter with extensive polygonal fracturing, whereas the pyroxene-bearing caprock (green) is partially covered by ripples and appears hilly and uneven in topography due to differential erosion. The textures of the PHS- and FeSO4OH-bearing units are distinct from those of the pyroxene-bearing units, but appear related to each other with fine-scale layering that varies in brightness, color, and fracturing.
The presence of highly pure FeSO4OH outcrops neighboring MHS at Aram Chaos and less pure outcrops of FeSO4OH neighboring PHS on the plateau NW of Juventae Chasma indicate an active geochemical history in Mars’ past. The hydrated sulfates likely formed in evaporative environments, while the FeSO4OH likely formed through heating. The FeSO4OH-bearing units at the Juventaue plateau could be mixed with spectrally neutral components that dilute the FeSO4OH spectral features. Coordinated characterization of the near-infrared (NIR) and mid-IR spectral features of Fe sulfates (Fig. 6) is enabling a better understanding of the spectral features due to FeSO4OH in these intriguing outcrops on Mars.
Acknowledgements: The authors are grateful for support from NASA MDAP #80NSSC21K1103, NASA SSW #80NSSC23K0032, Austrian Science Fund FWF #P34227-N, and Europlanet Transnational Access funds.
References: [1] Bishop J.L. et al. (2009) Mineralogy of Juventae Chasma…, JGR, 114, doi:10.1029/2009JE003352. [2] Lichtenberg K. A. et al. (2010) Stratigraphy of hydrated sulfates in the sedimentary deposits of Aram Chaos, Mars, JGR, 115, doi:10.1029/2009JE003353. [3] Bishop J. L. et al. (2024) Characterizing the spectral properties of a new FeSO4OH phase observed on Mars, LPSC, #1880. [4] Meusburger J. M. et al. (2024) Ferric hydroxysulfate on Mars and its formation from ferrous sulfate hydrates, 10th Mars Conference. [5] Itoh Y. & M. Parente (2021) A new method for atmospheric correction and de-noising of CRISM data, Icarus, 354, 114024. [6] Saranathan A.M. & M. Parente (2021) Adversarial feature learning for improved mineral mapping of CRISM data, Icarus, 355, 114107. [7] Yeşilbaş M. et al. (2024) Low-temperature reflectance spectra of szomolnokite and applications for their detection on Mars, LPSC, #2035.
How to cite: Bishop, J. L., Meusburger, J. M., Talla, D., Weitz, C. M., Parente, M., Gross, C., Saranathan, A. M., Itoh, Y., Gruendler, M. R. D., Howells, A. E. G., Yeşilbaş, M., Hiroi, T., Schmitt, B., Maturilli, A., Al-Samir, M., Bristow, T. F., Lafuente, B., and Wildner, M.: Characterizing the New Mineral FeSO4OH on Mars and Describing its Geochemical History and Association with Other Sulfates , Europlanet Science Congress 2024, Berlin, Germany, 8–13 Sep 2024, EPSC2024-289, https://doi.org/10.5194/epsc2024-289, 2024.
Introduction
The Emirates Mars InfraRed Spectrometer (EMIRS) instrument onboard the Emirates Mars Mission (EMM) “Hope” probe is a Fourier Transform Infrared spectrometer that has been observing the Martian surface and atmosphere between 6 and 100 μm since February 2021 [1, 2]. The unique orbit of EMM allows EMIRS to observe the entire Martian disk at each observation, covering all the surface of the planet in ~4 orbits, which corresponds to ~5° of Ls, or 10 Earth days.
[3] used the surface temperature retrievals from EMIRS to detect and monitor the presence of H2O and CO2 ice on the surface of the planet, including the temporal and local time evolution of the CO2 frost that can appear at Martian equatorial latitudes. This frost has been observed in the second half of the night around the equinoxes. Small crystals and optically thin layers are expected from the work of [4] using THEMIS data. However, as EMIRS provides fully resolved spectra whereas THEMIS is a multi-band instrument, we will be able to strengthen the constraints on the physical properties of these deposits. And monitor for the first time the evolution of the ice properties during its condensation phase from 12 a.m. to 6 a.m. thanks to the unique temporal coverage of EMM instruments.
Data & Methods
Based on the first identification of the EMIRS pixels mostly covered by CO2 ice presented in [3], we use here the full spectroscopic power of the instrument to characterize and constrain the physical properties of these icy deposits (crystal size, thickness) and their temporal evolution.
First, we identify areas where CO2 surface frost has been detected at different local times over a few degrees of Ls(to have a sufficient spatial and temporal coverage), then we bin the data over temporal bins of 1-hr and compare the averaged spectra for each bin. The spatial extent of the EMIRS pixel footprints is computed using the SPiP Python module [5].
Results
First, we observe that CO2 ice spectral signatures are observed in the EMIRS spectra when predicted by the temperature criterion, between midnight and 6 a.m., but mostly from 3 a.m. Comparison of spectra presented in Figure 1a with models from [4] may suggest optically thin layers of CO2 ice, with a thickness of ~ 100 μm, when models can predict condensation of up to a few tens of microns of ice just before sunrise.
As the surface temperature remains below the freezing point of CO2 during the second half of the night, and CO2 is always available at the surface from the atmosphere, condensation is expected to occur over the night hours. However, no significant temporal variations of the spectra have been observed over the night. The differences between spectra that can be seen in Figure 1 are mostly associated with spatial variability of the surface emissivity, as can be seen on panels c & d. This is not in favor of the scenario of an accumulation of ice over the night which would increase progressively the frost thickness and/or the size of the crystals. Thus, the lack of variability over the night may suggest that the condensation may occur within the surface regolith and/or the subsurface, as suggested by [6].
Figure 1: EMIRS spectra from EMM orbits 171 to 180 where surface CO2 frost has been detected between 150°W and 90°W and between 0°N and 60°N (North of Tharsis), averaged over temporal bins of 1-hr (a) and footprints of the considered EMIRS pixels (b). The colors of the spectra and pixels indicate the local time range for the observations. (c & d) EMIRS average spectrum and pixel footprints over the same region for observations between 11 a.m. and 1 p.m. to provide a daytime emissivity reference to be compared with the nighttime spectra.
Conclusion & Perspectives
In this work, we present the first spectroscopic monitoring of surface nighttime CO2 frost under equatorial latitudes as a function of the local time. We do not observe significant variation of the spectra over the night but a spatial variability is present. This suggests that the frost condensation likely occurs within the regolith and/or close subsurface rather than a simple accumulation of ice at the surface overnight. Further spectral modeling of the emissivity will be needed to assess the physical properties of these deposits considering different scenarios including notably: “dirty” CO2 ice, subsurface CO2 ice, or slab of CO2 ice.
Acknowledgments
This work was funded by the Emirates Mars Mission project under the Emirates Mars Infrared Spectrometer instrument via The United Arab Emirates Space Agency (UAESA) and the Mohammed Bin Rashid Space Centre (MBRSC).
A. S. also acknowledges funding by CNES.
References
[1] Amiri, H. E. S. et al. (2022), SSR, 218, 4. [2] Edwards, C. S. et al. (2021), SSR, 217, 77. [3] Stcherbinine, A. et al. (2023), GRL, 50, e2023GL103629. [4] Piqueux, S. et al. (2016), JGR: Planets, 121, 1174-1189. [5] Stcherbinine, A. (2023), Zenodo, doi:10.5281/zenodo.7714204. [6] Lange, L. et al. (2022), JGR: Planets, 127, e2021JE006988.
How to cite: Stcherbinine, A., Edwards, C., Haberle, C., Smith, M., Lange, L., and Pilorget, C.: Spectroscopic Characterization and Evolution of Martian Nighttime CO2 Frost at Equatorial Latitudes with EMM/EMIRS, Europlanet Science Congress 2024, Berlin, Germany, 8–13 Sep 2024, EPSC2024-50, https://doi.org/10.5194/epsc2024-50, 2024.
INTRODUCTION
Dome-shaped uplifted and fractured terrain has been observed at the surface of volcanic regions on Mars, amongst other terrestrial planets and moons in our Solar System. Such dome-shaped ground deformation features include, for example, domes in Elysium Planitia and floor-fractured craters (e.g., Farrand et al., 2011; Jozwiak et al., 2012). These features are inferred to have formed by the emplacement and inflation of sill- and laccolith-shaped magma bodies in the shallowest 1-2 km of the crust (e.g., Michaut et al. 2013). This interpretation is informed by monitored magma intrusion events and geological observations at eroded outcrops of volcanic plumbing systems on Earth. On Mars and other planetary bodies, however, only the final surface deformation features can be observed from orbit. Besides studying analogue outcrops on Earth, analytical and numerical models are used instead to understand the emplacement dynamics and the deformation of the planetary crust´s rocks. A mismatch exists, however, between the oversimplified intrusion geometry and the linearly elastic response to magma intrusion assumed by most numerical models, and the complex intrusion geometries and mechanical response of host rocks to magma-induced stresses observed on Earth in exposed volcanic plumbing systems. Strain can accumulate along large-scale discontinuities in the overburden rocks, making the investigation of the emplacement mechanisms by traditional continuum models difficult. To investigate the ill-understood effect on magma-induced deformation of the lower gravity on Mars, and the Moon, due to their smaller mass compared to Earth, we compare simulations of laccolith inflation in the Discrete Element Method (DEM) under gravity of the Moon, Mars and Earth.
METHOD
We used the two-dimensional (2D) DEM particle flow code PFC2D (Itasca Consulting Group, Inc.) to simulate the inflation of a half-ellipsoid laccolith in a rock medium represented as a particle-based assemblage. Previously, we have shown how this model can indicate fracturing and highly discontinuous deformation, as well as visualise the localization of subsurface strain and corresponding deformation (for details see Morand et al., 2024). To simulate laccolith inflation, the laccolith-shaped pressure source is inflated by increasing the area of the 2D particles (Figure 1). We ran inflation simulations in rocks of different toughness and stiffness representing a range of realistic crustal strengths, under the gravity of the Moon (g = 1.62 m.s-2), Mars (g = 3.71 m.s-2), and Earth (g = 9.81 m.s-2).
Figure 1. General view of the DEM model in PFC2D with rock particles (grey) and the initial particle-based, laccolith-shaped magmatic body (red) at 1 km depth. The subsets display the magma reservoir area in its initial state before inflation and in its final state after 25% of inflation.
RESULTS AND DISCUSSION
For equal rock stiffness and amounts of intruded magma, our model results show that we can expect the same volume of magma injected at the same depth to induce more vertical surface displacement on Mars, due to the lower gravity there compared to Earth. Nevertheless, rock toughness and rock stiffness control the amount of fracturing more than gravity does. These findings are even better expressed under Lunar gravity. Our finding implies that both gravity and realistic crustal rock strength are essential parameters to account for in modelling efforts of magma-induced deformation on moons and planets such as Mars with a size and mass that is significantly different from that of Earth.
Our model results will induce a better understanding of the emplacement and architecture of shallow magmatic intrusions below magma-induced uplifted terrain and floor-fractured craters on Mars. Verification of this new modelling application with detailed structural information from analogue magma intrusions on Earth, such as Permian trachy-andesite intrusions in the Intra-Sudetic Synclinorium in SW Poland, allow us to investigate the characteristics of the cryptic magma bodies underlying surface doming on Mars and the Moon, while simultaneously improving the interpretation of volcanic unrest signals on Earth.
REFERENCES
Farrand, W.H. et al. (2011) “Spectral evidence of volcanic cryptodomes on the northern plains of Mars.” Icarus, 211(1), 139–156. https://doi.org/10.1016/j.icarus.2010.09.006
Jozwiak, L.M. et al. (2012) “Lunar floor-fractured craters: Classification, distribution, origin and implications for magmatism and shallow crustal structure.” Journal of Geophysical Research E: Planets, 117(11), 1–23. https://doi.org/10.1029/2012JE004134
Michaut, C., et al. (2013) “Magmatic intrusions and deglaciation at mid-latitude in the northern plains of Mars.” Icarus, 225(1), 602-613. https://doi.org/10.1016/j.icarus.2013.04.015
Morand, A. et al. (2024) “Fracturing and dome-shaped surface displacements above laccolith intrusions: Insights from Discrete Element Method modeling.” Journal of Geophysical Research: Solid Earth, 129, e2023JB027423. https://doi.org/10.1029/2023JB027423
How to cite: Poppe, S., Cornillon, A., Morand, A., Harnett, C. E., and Mège, D.: Displacement and fracturing around laccolith intrusions affected by gravity on Mars versus the Moon, and Earth, Europlanet Science Congress 2024, Berlin, Germany, 8–13 Sep 2024, EPSC2024-761, https://doi.org/10.5194/epsc2024-761, 2024.
Introduction
Sublimation experiments performed in vacuum chambers have proven useful for understanding the processes that occur on the surfaces of volatile-rich celestial bodies without significant atmospheres [1, 2, 3]. To date, these experiments have focused on the sublimation of volatile ices without considering the possibility of a liquid phase being present. The most prominent example is Mars, the surface of which appears as an ultra-arid cold desert nowadays, but the presence of liquid water in its past is essentially assured [4, 5].
On Mars, impact events or seasonally increased insolation might locally thaw near-surface permafrost and release liquid water, even long after transitioning from a more humid to an ultra-arid and low-pressure environment. The liquid water could solute and transport salts away from one location to another, where they could form deposits after the eventual evaporation of the water.
Suppose salt crusts or related morphological features of the Martian surface can be reproduced in sublimation chambers using saline solutions and regolith analogues. This would shed light on how salt deposits on Mars formed after the planet lost most of its atmosphere.
Scientific objectives are:
- What physical environmental parameters and chemical composition affect the sustainability of surface-near aqueous solutions in a cold, low-pressure environment?
- Does liquid water sublimate from the sample surface and form salty crusts or efflorescences?
- Do saline ices and brines form near-surface features such as ice lenses?
Methods
In a vacuum sublimation chamber, environmental conditions on the Martian surface were reproduced, and the conditions under which saline solutions can migrate through a regolith analogue were investigated. The focus was on the parameters of salt composition and concentration, as well as the grain size of the regolith analogue. In particular, Mg2+, Fe3+, Cl-, and SO42- based salts were used since they are observed in specific areas of the Martian surface [6, 7].
Mars regolith analogues were saturated with brines and placed in a transparent sample container in a sublimation chamber. The environmental conditions in the chamber were adapted to the Martian summer and account for 243 K temperature, 5 mbar atmospheric pressure, and 600 Wm-2 irradiance.
Each experiment lasted several days, during which the lateral and horizontal sample surfaces were permanently monitored with cameras. After completing the sublimation experiments, the sample's internal layer structure was analysed. The reflectance of each sample surface was measured in the spectral range of 400-2500 µm. Eventually, the cone tip penetration method was used to test the resistance of the surface to mechanical stress.
We carried out experiments on two scenarios with different salt concentrations, in which the grain size of the regolith analogue was varied at low and high salinity. The used grain sizes were 212-500 µm, 1000-2000 µm, and 212-2000 µm.
Results
After the sublimation experiments, the samples generally presented four different layers (Fig. 1).
- A solidified crust formed on the surface. The thickness of the crust was determined by the grain size, whereby the thicknesses increased with increasing grain sizes. With increasing grain size, the crust's cone tip resistance (CTR) was reduced by an order of magnitude, respectively. However, the resistance to mechanical stress also depended strongly on the salinity. For the same grain size, the CTR for the high salinity scenario was about one magnitude higher than in the low salinity scenario. Analyses of the reflectance spectra showed a clear blue slope of salt-rich surfaces, which became more distinct with decreasing regolith grain size (Fig. 2). However, no pure salt deposits could be produced on the surface, even in the high salinity scenario.
- Below the crust, a layer of dry, unconsolidated material formed. Notably, in the samples of the low salinity scenario, salt crystallised and gave the layer a bright appearance.
- Then, a slightly moist layer followed, and dark-yellowish crystals formed. The thickness of the layer was comparable for both salinities and only increased slightly with increasing grain size.
- The bottom layer was moist with brines and contained considerable quantities of water ice crystals. The depth at which this layer formed was determined by grain size, not salinity. Small grain sizes favoured the formation of larger ice crystals, which grew to 5 mm in size. The ice crystals were clear and had a lower salt content compared to the initial solution.
Fig 1: Schematic representation of the resulting layers after completion of the sublimation experiments. The camera images show examples of the layers with various grain sizes and salinities.
Fig 2: Reflectance spectra of sample surfaces with a regolith grain size of 212-500 µm. With increasing salinity of the sample, the spectra show a distinct blue slope.
References
[1] Poch, O., et al. 2016, Icarus, 267, 154-173. doi:10.1016/j.icarus.2015.12.017
[2] Mc Keown, L.E., et al. 2017, Sci Rep 7, 14181. doi:10.1038/s41598-017-14132-2
[3] Haack, D., et al. 2021, Astronomy & Astrophysics, 649, A35. doi:10.1051/0004-6361/202140435
[4] Irwin III, R.P., et al. 2004, Journal of Geophysical Research: Planets, 109(E12). doi:10.1029/2004JE002287
[5] Wray, J., 2021, Annual Review, 49, 141-171. doi:10.1146/annurev-earth-072420-071823
[6] Mangold, N., et al. 2008, Icarus, 194, 519-543. doi:10.1016/j.icarus.2007.10.021
[7] Osterloo, M.M., et al. 2008, Science, 319(5870), 1651–1654. doi:10.1126/science.1150690
How to cite: Haack, D., Kaufmann, E., and Hagermann, A.: Evolution of brines in regolith analogue under simulated Martian surface conditions, Europlanet Science Congress 2024, Berlin, Germany, 8–13 Sep 2024, EPSC2024-85, https://doi.org/10.5194/epsc2024-85, 2024.
Please decide on your access
Please use the buttons below to download the supplementary material or to visit the external website where the presentation is linked. Regarding the external link, please note that Copernicus Meetings cannot accept any liability for the content and the website you will visit.
Forward to presentation link
You are going to open an external link to the presentation as indicated by the authors. Copernicus Meetings cannot accept any liability for the content and the website you will visit.
We are sorry, but presentations are only available for users who registered for the conference. Thank you.
Mars offers abundant raw materials that are of potential value for future human endeavors. Numerous vital elements can be found directly at the surface. The exploration of these natural resource deposits, the concentration of the raw materials contained in them, and the feasibility of mining and refinement are open questions that require further consideration. The natural geological variations in ore deposits can significantly impact viability of exploration sites and of the engineering architecture. Therefore, comprehension of these features is crucial for selecting and optimizing a particular technical design. This underlines the need for accurate resource exploration missions. Refinement and restructuring must be investigated to provide reliable fabrication systems since In Situ Resource Utilization (ISRU) is able to drastically cut down logistical dependence from Earth.
Water is an essential requirement for an extended stay on Mars. It will serve as a consumable for astronauts and is needed for the production of propellant and oxygen, and for construction use. Water could even serve as radiation shielding if procurable in sufficient amounts. In contrast to subterranean ice, hydrated minerals offer a promising and reliable potential for water extraction directly at the surface of Mars and at lower latitudes. A large diversity of hydrated minerals such as phyllosilicates, hydrated silica, zeolites, and sulfates have been detected from orbit using visible near infrared (VIS-NIR) reflectance spectroscopy [1-3]. We address the concept of harvesting resources in situ by reviewing the potential of several large deposits of hydrated minerals found on the surface of Mars thanks to previous orbital detections (Fig. 1). We also present estimates of water volumes potentially retrievable from specific deposits and describe additional uses of the hydrated minerals for example as feedstock for a Bio-regenerative Life-Support System (BLSS).
A BLSS architecture tends to be superior in terms of mass efficiency compared to non-bio-regenerative, i.e., chemical or physical life support systems, especially for protracted mission durations [4]. BLSS have the unique advantage of managing biological waste through biological processes exclusively. In addition, if higher plants are cultivated, they offer psychological comforts and nutritional benefits derived from the presence of plant life and as fresh food [5]. Thus, BLSS systems realized in the form of one or multiple greenhouses, are an indispensable component for sustaining a human presence on Martian terrain [6, 7]. Over the last 40 years, several studies have shown that crops can be efficiently grown on hydroponic or soilless media [8, 9]. In fact, crop cultivation in controlled environments can even meet or surpass record yields [10]. From 2018 to 2022, the EDEN ISS project from the German Aerospace Center operated a space-analog test facility greenhouse near the Neumayer III station in Antarctica, potentially resulting in the closest Mars analog we can find on Earth (Fig. 2). In the first year of operation, 268 kg of edible biomass was produced on the 12.5 m2 cultivation area of the greenhouse [11] with soilless media. Additional advantages include removing CO2 from the atmosphere, keeping water in a recycling loop, and producing oxygen. These findings demonstrate the importance of BLSS and can provide a scalable estimate of the potential contribution to logistics and required transport of materials for long duration crewed missions.
- [1] S.L. Murchie et al. (2009). A synthesis of Martian aqueous mineralogy after 1 Mars year of observations from the Mars Reconnaissance Orbiter, J Geophys Res Planets 114. https://doi.org/10.1029/2009JE003342.
- [2] J.F. Mustard et al. (2008). Hydrated silicate minerals on Mars observed by the Mars Reconnaissance Orbiter CRISM instrument, Nature 454, 305–309. https://doi.org/10.1038/nature07097.
- [3] B.L. Ehlmann, C.S. Edwards (2014). Mineralogy of the Martian Surface, Annu Rev Earth Planet Sci 42, 291–315. https://doi.org/10.1146/annurev-earth-060313-055024.
- [4] C. Lasseur (2010). Melissa: The European project of a closed life support system, Gravitational and Space Biology 23 (2).
- [5] C. Mitchell (1994). Bioregenerative life-support systems, Am J Clin Nutr, 60 820S-824S. https://doi.org/10.1093/ajcn/60.5.820S.
- [6] M. Bamsey et al. (2009). Canadian advanced life support capacities and future directions, Advances in Space Research 44, 151–161. https://doi.org/10.1016/j.asr.2009.03.024.
- [7] H. Liu et al. (2021). Review of research into bioregenerative life support system(s) which can support humans living in space, Life Sci Space Res (Amst) 31, 113–120. https://doi.org/10.1016/j.lssr.2021.09.003.
- [8] J.F. Thomas, C.D. Raper (1983) Photoperiod Effects on Soybean Growth during the Onset of Reproductive Development under Various Temperature Regimes, Botanical Gazette 144, 471–476. http://www.jstor.org/stable/2474450.
- [9] Y. Tako (2001). Integration of Sequential Cultivation of Main Crops and Gas and Water Processing Subsystems Using Closed Ecology Experiment Facilities, https://doi.org/10.4271/2001-01-2133.
- [10] R.M. Wheeler, T.W. Tibbitts (1987). Utilization of potatoes for life support systems in space: III. Productivity at successive harvest dates under 12-H and 24-H photoperiods, Am Potato J 64, 311–320. https://doi.org/10.1007/BF02853523.
- [11] P. Zabel et al. (2020). Biomass Production of the EDEN ISS Space Greenhouse in Antarctica During the 2018 Experiment Phase, Front Plant Sci 11. https://doi.org/10.3389/fpls.2020.00656.
Figure 1: Color-coded Mars Orbiter Laser Altimeter (MOLA) map showing the example regions in dashed white box.
Figure 2: The EDEN ISS greenhouse in Antarctica with the Neumayer III station in the background (top). Cultivation area inside the test container (bottom).
How to cite: Gross, C., Al-Samir, M., Bishop, J. L., Poulet, F., Postberg, F., and Schubert, D.: Resource Reconnaissance for Future Crewed Missions to Mars , Europlanet Science Congress 2024, Berlin, Germany, 8–13 Sep 2024, EPSC2024-807, https://doi.org/10.5194/epsc2024-807, 2024.
The Mars Express Radio Science Experiment (MaRS) [1] performs bistatic radar measurements (BSR) every two years during the opposition between Earth and Mars when the distance between them is smallest. In 2022, three measurements were successfully executed at the end of November and December over different regions of Mars.
The dielectric properties and surface roughness of Mars can be determined using BSR measurements [2, 3]. In this process, the radio subsystem transmitter sends right circularly polarized (RCP) radio signals at two wavelengths, X-Band and S-Band, toward Mars' surface. Part of the transmitted radiation is then scattered back toward a receiver at a ground station on Earth. Both the right and left circularly polarized echo components (RCP and LCP, respectively) are recorded at the ground station.
The dielectric constant can be derived from the RCP-to-LCP power ratio, eliminating the need for absolute end-to-end calibration. Instead, relative calibration of the RCP and LCP ground receiver channels can be used. However, achieving accurate relative calibration of the two receiving channels remains challenging.
To improve the calibration procedure, an additional step has been added. This step involves pointing the 70 m ground station toward a radio source during the post-calibration process. A comparison between the old and new calibration procedures will be presented, along with initial results for the dielectric constant from all three measurements conducted in 2022.
References
[1] M. Pätzold et al., “Mars Express 10 years at Mars: Observations by the Mars Express Radio Science Experiment (MaRS),” Planetary and Space Science, vol. 127, pp. 44–90, Aug. 2016.
[2] R. A. Simpson, G. L. Tyler, M. Pätzold, and B. Häusler, “Determination of local surface properties using Mars Express bistatic radar,” Journal of Geophysical Research (Planets), vol. 111, no. #E10#, p. 6, 2006.
[3] R. A. Simpson, G. L. Tyler, M. Pätzold, B. Häusler, S. W. Asmar, and A. K. Sultan-Salem, “Polarization in Bistatic Radar Probing of Planetary Surfaces: Application to Mars Express Data,” Proceedings of the IEEE, vol. 99, no. 5, pp. 858–874, 2011.
Acknowledgements
The Mars Express Radio Science Experiment (MaRS) is funded by the Deutsches Zentrum für Luft- und Raumfahrt, Bonn-Oberkassel, under grants 50QM1004 and 50QM1005. We thank the ESA Mars Express Science Operations teams, the MEX Flight Control Team, and the NASA Deep Space Network for their support. Special thanks to the NAIF group at JPL, headed by Charles Acton, for their assistance with the SPICE software package.
How to cite: Scholl, D., Andert, T., Pätzold, M., and Semaan, A.: Bistatic Radar Observations in 2022 with Mars Express, Europlanet Science Congress 2024, Berlin, Germany, 8–13 Sep 2024, EPSC2024-487, https://doi.org/10.5194/epsc2024-487, 2024.
Abstract
MarsSI (“Mars Système d’Information”, French for Mars Information System) is a service to explore, request, process and retrieve planetary surface data https://marssi.univ-lyon1.fr/MarsSI.
1 Introduction
Geological investigations of planetary surfaces require the combination of orbital datasets. Missions being often multiple-instruments platforms from multiple space agencies, the quantity of data available increased quickly. It is now large enough that a dedicated system has to be used to explore, calibrate, process and retrieve the relevant information.
MarsSI [1] is a platform developed in the context of the e-Mars project (2012-2017) to facilitate the use of combined orbital data. It was certified in 2017 as french national Research Infrastructure by the Centre National de la Recherche Scientifique (CNRS) as part of the Planetary Surface Portal (PSUP) [2]. The permanent staff currently consists of one scientist lead and one engineer.
2 Architecture
Hosted by ENS-Lyon, MarsSI hardware consists of a frontend server, a storage bay a storage bay and a 80 core cluster. For the software stack, we decided to rely mainly on software provided by the FOSS communities and standard and documented protocols such as those proposed by the Open Geospatial Consortium (http: //www.opengeospatial.org/), managed in a PostGIS (https://postgis.net/) database.
3 Catalog
As of 2024, MarsSI indexes and give access to optical data (visible, multi and hyper-spectral) and derived products from three missions: Mars Odyssey, Mars Express and Mars Reconnaissance Orbiter. Figure 2 shows all the referenced products and the processing that can be applied to them. Our emphasis was to provide ”ready-to-use” products in regards of calibration, corrections and georeferencing. The user will be able to visualize and interpret the data in GIS or remote sensing software.
3.1 Imagery
MarsSI provides access to various optical datasets for visible, multi- and hyper-spectral data from the various martian orbital missions over the years. For example, for the MRO mission, CTX and HiRISE images are calibrated and map projected using the ISIS software suite (https://isis.astrogeology.usgs.gov/). CRISM hyperspectral data is processed using the CRISM Analysis Toolkit (CAT) pipeline developed by the CRISM team [3][4]. Spectral cubes are calibrated to I/F and corrected from the atmospheric contribution with the volcano-scan method [5] and spectral parameter maps are produced. This procedure was also applied to the OMEGA dataset.
3.2 DEMs
We offer multiple Digital Elevation Model (DEM) datasets in MarsSI. Some of them are provided from external sources (such as those provided by the HiRISE and HSRC teams). But users can also requests med- and high- resolution DEMs generated using the Ames Stereo Pipeline software [6]. Possible DEMs are computed by analyzing the CTX and HiRISE coverage footprints to find image pairs with overlapping by at least 60% and a minimum deviation of 10° in emission angle. While these products are the results of automated procedures and cannot benefit from human quality control and fine tuning, they do provide much larger coverage of Mars surface: HiRISE team provided DEMs cover 0.04% of Mars surface, while MarsSI HiRISE-based DEMs cover 3.84% and CTX-based DEMs 16.93%. The DEM generation workflow was updated in 2020 with a completely new version[7].
4 User interface
MarsSI client interface is a web application that aims to be as straightforward as possible and received many updates in the previous years, starting with a complete rewrite. As shown on figure 3, the user can explore the datasets over a map interface. Data can be selected and sent to a workspace. The workspace view, shown on figure 4, allow to review products in detail. This is also where users will be able to request dataset processing. User can create more workspaces to organize their data selections.
When datasets processing are finished, the user will request a copy operation. The selected data will be copied to a directory that is aviable through SFTP using the user credentials. Files in that directory are kept for 30 days.
5 Documentation
A wiki has been set to offer tutorials, with data specific pages including brief data descriptions and relevant links. A introduction video tutorial is available. In 2023, a ticketing system was also launched to report issues.
6 User community
MarsSI is open to the scientific communities around the world. As of april 2024, we count more than 600 registered users. Naturally for french service, 25% of the users are from France, but we also offer data to scientists from the USA, UK, India and China.
7 Perspectives
After many improvements to the platform, we now aim to complement our datasets. We are adding the SHARAD catalog and aim to provide a service to create radargram simulation based on our CTX DEM dataset to help interpret the real data. We also expect to expand the service to the Moon next year and provide LROC based datasets.
8 Conclusion
Built upon opensource frameworks and using standardized protocols, MarsSI offers the scientific communities a way to explore space agencies catalogs and automatically process them to high value products, notably DEMs derived from CTX and HiRISE datasets and spectral parameter maps from CRISM and OMEGA datasets.
Acknowledgments
MarsSI is part of national Research Infrastructure PSUP, recognized as such by the French Ministry of Higher Education and Research under the ANO5 label. It was supported by the Programme National de Planétologie (PNP) of CNRS/INSU, co-funded by CNES.
9 References
[1] C. Quantin-Nataf et al. In: Planetary and Space Science 150 (2018). [2] F. Poulet et al. In: Planetary and Space Science 150 (2018). [3] S. Pelkey et al. In: Journal of Geophysical Research: Planets 112.E8 (2007). [4] S. Murchie et al. In: Journal of Geophysical Research: Planets 112.E5 (2007). [5] P. C. McGuire et al. In: Planetary and Space Science 57.7 (2009). [6] Z. M. Moratto et al. In: Lunar and Planetary Science Conference. Vol. 41. 2010. [7] M. Volat, C. Quantin-Nataf, and A. Dehecq. In: Planetary and Space Science 222 (2022).
How to cite: Volat, M., Quantin-Nataf, C., Mandon, L., Torres, I., and Millot, C.: MarsSI: Martian surface data processing service , Europlanet Science Congress 2024, Berlin, Germany, 8–13 Sep 2024, EPSC2024-49, https://doi.org/10.5194/epsc2024-49, 2024.
Introduction:
Chloride deposits on Mars serve as important mineralogical markers for the planet’s dynamic aqueous past, recording the last phase(s) of liquid water activity (e.g., [1-3]). They have often been considered as evidence of extensive reservoirs of surface/subsurface groundwater on early Mars, facilitating chemical sedimentation and perhaps serving as sites for potential biological activity. Most previous campaigns to detect and characterize chloride-bearing deposits have been based on their distinctive thermal infrared spectral signatures [1-2]. More recently, efforts have also been made to employ machine learning (ML) algorithms to identify “potential chloride-bearing” deposits [4], although validation of such detections has been challenging. This study explores the VNIR spectral characteristics of chlorides, and the capability of currently operational VNIR instruments like the High Resolution Stereo Camera (HRSC) [5], the Colour and Stereo Surface Imaging System (CaSSIS) [6] and the High Resolution Imaging Science Experiment (HiRISE) [7] to spectrally identify/characterize chlorides on Mars, with an aim to potentially serve as a way to validate recent ML-based detections [4].
Methods:
In this study, we employ previously utilized and well-known methods like spectral shape and spectral parameter analysis (e.g., [8-11]) to assess the chloride-detection capability of HRSC, CaSSIS, and HiRISE. Prior to spectral analysis, an empirical dark-subtraction correction (e.g., [8-11]) is also applied to the radiometrically calibrated products to mitigate effects of atmospheric scattering. While this abstract outlines preliminary results from CaSSIS, where applicable, similar inferences may be made for HRSC and HiRISE.
Results and Discussions:
VNIR Spectral Characteristics of Chlorides:
Chlorides generally do not possess any diagnostic features in the VNIR [12-13]. Despite that, their VNIR spectral behaviour is still distinctly different from other commonly occurring Martian minerals, as they remain one of the few minerals along with kaolinite and hematite that do not possess a negative IR slope characteristic of dust/mafic mixtures. Fig. 1 shows plots of laboratory-based reference spectra [14] of hematite, kaolinite and halite and the corresponding spectral response resampled to HRSC, CaSSIS and HiRISE wavelengths. Theoretically, at HRSC and CaSSIS wavelengths, it should be possible to distinguish hematite from kaolinite and chloride-bearing deposits (Fig. 1). Alternatively, kaolinite and chloride exhibit similar spectral behaviour, although the RED-IR/RED-NIR slopes for chloride (Fig. 1c) are generally steeper than that for kaolinite (Fig. 1b). At HiRISE wavelengths however, the three minerals lack distinguishability from each other due to the limited sensitivity of HiRISE band-passes to the RED-IR spectral characteristics of these materials.
Appearance in Colour and Spectral Band Ratio Composites:
In panchromatic images, like those from the Context Camera [15], chloride-bearing deposits often appear bright and are indistinguishable from other light-toned deposits. However, they show a distinct behaviour in colour infrared multispectral observations. Chlorides have been observed to have a pink-purple hue in colour composites images (e.g., [1,16]). Fig. 2 shows snapshots of four colour composite combinations (NIR-PAN-BLU, RED-PAN-BLU, NIR-RED-BLU and NIR-RED-PAN) of three CaSSIS images showing chloride (Figs. 2a-2d), kaolinite (Figs. 2e-2f) and hematite-bearing (Figs. 2i-2l) deposits respectively. In most CaSSIS colour combinations, the colour of the kaolinite-bearing deposit (Figs. 2e-2g) appears pink like that of chloride (Figs. 2a-2c). However, the steeper RED-NIR slope observed for chlorides in comparison to kaolinite causes them to be distinguishable in NIR-RED-PAN colour composites, with kaolinites appearing pale yellow (Fig. 2h) and chlorides appearing relatively pale pink (Fig. 2d). Hematite bearing deposits, on the other hand, appear relatively brown in all four colour composites, allowing them to be distinguished easily from kaolinites and chlorides.
Since Mars' surface is predominantly covered with dust rich in ferric iron, the effects of the absence of a significant IR slope are further accentuated in the band-ratio space, resulting in distinct appearances of chlorides in colour band ratio composite (CBRC) images (Fig. 3). Chlorides typically appear prominently red in CBRCs built by assigning the IR/RED, RED/BLU, and RED/IR spectral ratios to the red, green and blue display channels, respectively. This colour differentiation arises from the positive RED-IR slope of chlorides (Fig. 1), which enhances the IR/RED ratio while significantly reducing the value of the RED/BLU spectral parameter. Such CBRCs may also enable global-scale mapping (e.g., [4]) at resolutions better than previous chloride surveys [2].
Spectral Shape Analysis:
It is also observed that performing four-point spectral analyses on DS-corrected CaSSIS images can also serve as means to validate ambiguous chloride deposits. This is described in Fig. 4 where it is shown that the use of the correction results in arriving at a very close match to the reference halite spectrum in Fig. 1(c). Consequently, this shows that a combination of the spectral methods can provide an efficient means of verifying small-scale deposits with reasonable accuracy.
Conclusions:
Our preliminary results show that despite chlorides lacking any VNIR diagnostic absorptions, their unique spectral characteristics allow for them to be characterized with reasonable confidence from multispectral instruments like CaSSIS. While similar spectral characteristics between kaolinite and chlorides are observed, usage of a combination of colour composite maps, CBRCs and spectral shape analysis may aid in separation between the two materials. Integrating these results with topographic information and general geological context may serve as an effective way to enable confident VNIR spectral detection of chloride-bearing deposits, which may be particularly useful to validate results from ML-based surveys [4].
Acknowledgements:
The lead author acknowledges support from LLT's funding from the CSA's Planetary and Astronomy Missions Co-I programme (22EXPCOI3) and the Canadian NSERC Discovery Grant programme (RGPIN 06418-2020).
References:
[1] Osterloo et al. (2008). Science, 319(5870). [2] Osterloo et al. (2010). JGR, 115(E10), E10012. [3] Leask and Ehlmann (2022). AGU Advances, 3(1).[4] Bickel et al. (2023). LPSC LIV, p.1478.[5] Jaumann et al. (2007). PSS, 55(7-8). [6] Thomas et al. (2017). SSR, 212. [7] McEwen et al. (2007). JGR, 112(E5).[8] Tornabene et al. (2018). SSR, 214.[9] Tornabene et al. (2022). LPSC LIII, p.2330.[10] Rangarajan et al. (2023a). Icarus, p.115443.[11] Rangarajan et al. (2023b). Icarus, p.115849.[12] Murchie et al. (2009). JGR, 114. [13] Viviano et al. (2014). JGR, 119.[14] Kokaly et al. (2017). USGS Spectral Library Version 7.[15] Malin et al. (2007). JGR, 112(E5).[16] Ye and Glotch (2019). JGR 124(2).
How to cite: Rangarajan, V. G., Tornabene, L. L., Hauber, E., Adeli, S., and Bickel, V.: Assessment of the sensitivity of VNIR multispectral datasets to detect chloride-bearing deposits on Mars, Europlanet Science Congress 2024, Berlin, Germany, 8–13 Sep 2024, EPSC2024-687, https://doi.org/10.5194/epsc2024-687, 2024.
- 1. Introduction
Fig. 1 Spider-like features or “spiders” on Mars, observed by remote sensing images
Atmosphere of Mars consists primarily of CO2 (96% by volume) [1, 2], up to 25% atmosphere undergoes a seasonal CO2 cycle of sublimation/condensation [1, 2]: in winter/autumn, atmospheric CO2 condenses as seasonal CO2 ice at the polar surface; in spring/summer, it sublimates back into the atmosphere. This seasonal CO2 cycle creates many exotic phenomena, e.g., dark spots and araneiform terrain or “spiders” (Fig. 1) [3-9]. Their formation process is believed as [3-9]: solar energy penetrates seasonal CO2 ice, later causing CO2 gas jetting and dust eruption onto the ice surface, forming dark spots. With yearly repetitions, spiders are formed showing radial or dendritic troughs. Spider formation is directly linked to seasonal CO2 cycling, thus Mars climate and an element in shaping the polar surface [10].
It is openly debated how climate affects spiders’ spatial configurations, geomorphologies and formation. To address these, sufficient spider observations are required. Currently the only spider observations are through vast remote sensing images as they have no earthly analogies. But this poses the following issue: how to effectively detect, then map spiders besides recruiting citizen scientists which possibly omits some spider observations due to understanding levels of spider formation.
This work focuses on applying super-detection capabilities of artificial intelligence (AI) for faster and more efficient detection of potential growth, spatial configuration and various morphologies of spiders based on remote sensing images, reducing uncertainties and low speed of human’s visual inspections which can be subjective, deepen our understanding of mechanisms behind spider formation.
- 2. Data and AI methods
We used HiRISE 5 Martian years images [11] at this stage. We tried to include an equal number of images for each type of spiders to prevent model bias.
Two types of AI methods were used in this work:
2.1 Conventional image processing techniques involve basic image manipulation such as image preprocessing to enhance contrasts. It uses local window binarization to highlight more intense pixels.
2.2 Deep learning with a single-stage detector applies “You Only Look Once” (YOLO, by Ultralytics) algorithm for object detection, which is more sophisticated suited for complex image recognition. To refine and enhance performance of YOLO detectors for spiders, several strategies are implemented, focusing on dataset improvement, model tuning, and advanced training techniques [12, 13].
a. Model Architecture and Parameter Tuning
Experiment with various YOLO Configurations, e.g., YOLOv8-Nano, Small, Medium and XLarge, help find the optimal balance between speed and accuracy for the detecting spiders task.
Hyperparameter Optimization are obtained by systematic testing of different hyperparameters, including learning rate, batch size, and number of epochs, to identify the best settings for the model.
b. Advanced Training Techniques
Transfer Learning is leveraged by starting with a model pre-trained on similar tasks and fine-tune it on the spider detection task. This significantly enhances the learning efficiency and final model performance.
Data Augmentation is expanded by incorporating random rotations, scaling, and other transformations to make the model robust to various spatial variations.
Multi-scale Training with images at multiple scales are to improve ability to detect various spiders.
- 3. Initial results and dissussion
Fig. 2 Results and confusion matrices for YOLO v8 size=nano (top), small (middle) and medium (bottom), with 50 (a and b) and 100 (c and d) epochs
Fig. 3 Predicted detection from the tunned deep learning model (left, Labels; right, predictions)
The method 1 had limited success in accurately detecting spiders due to the similar coloration of the spiders and their backgrounds and their complex, ramified structures. It is more effective for identifying dark spots, which are relatively distinct from their surroundings.
Figs. 2 and 3 show the method 2, the YOLO demonstrated superior performance in detecting spiders, benefiting from its ability to learn from the data and distinguish spiders from complex backgrounds. (1) Different configurations of YOLO (Fig. 2) were tested, with the smaller models (Nano, Small) providing a good balance between speed and accuracy, sufficient for the dataset used. (2) The model was effective even when spiders were not distinctly separable from the background, showing potential for identifying overlapping spiders.
The models were trained using various computational resources to optimize performance. Our results showed that extending training beyond 50 epochs did not significantly improve performance, likely due to the small size of dataset. Precision and recall were used as metrics, and the models achieved good results with relatively few false positives.
- 4. Conslusion
Primarily, we tested YOLO on the given images. Next, we plan to extend the implementation to more state-of-the-art AI approaches to evaluate the performances across multiple benchmarks. Such as, alternative deep learning models or more complex YOLO configurations which can be used to enhance detection accuracy. Overall, the deep learning approach, particularly YOLO, proved to be a promising method for the automated detection of Martian spiders, indicating a significant step forward in the application of AI technologies for planetary sciences.
References. [1]Leighton, Murray, 1966. Science 153. [2]Nier, McElroy, 1977. JGR 82. [3]Kieffer, 2007. JGR 112. [4] Piqueux et al. 2003. JGR 108 (E8). [5]Portyankina et al. 2017. Icarus 282. [6]Hansen et al. 2010. Icarus 205. [7] Hao et al. 2019. Icarus 317. [8] Diniega et al. 2021. Geomorphology 380. [9]Mc Keown et al. 2021. Sci Rep 11. [10]Piqueux, Christensen, 2008. JGR 113. [11] McEwen et al. 2007. JGR 112. [12] Hasan et al. 2021. Comput. Electron. Agric. 184. [13]Lodhi et al. 2022. ICRITO 2022 1-6
How to cite: Hao, J., Mokayed, H., Borg, L., Haseeb, S., and Schröder, S.: Catching "spiders" on Mars – investigation of Martian araneiform terrain by AI, Europlanet Science Congress 2024, Berlin, Germany, 8–13 Sep 2024, EPSC2024-127, https://doi.org/10.5194/epsc2024-127, 2024.
Abstract
Wind is one of the most important geological agents on today’s Mars and is responsible, for instance, for planet-wide migration of ripples and dunes. However, the significance of local topography for regional sand-moving wind patterns is still poorly understood. Here, we investigate the average turbulent surface wind shear stress over Gale Crater, Mars, by means of Computational Fluid Dynamics (CFD). Our results reveal how topographic effects at different scales affect local wind systems, thus contributing to understand present-day Aeolian activity throughout the crater. By coupling CFD to morphodynamic simulations of the sand landscape, we develop a numerical tool for modelling dune fields in Gale crater and in other Aeolian settings affected by strong local topography on Mars.
Introduction
The quantitative understanding of the genesis and dynamics of Martian dunes — which, in the Martian Sourthern Hemisphere, occur mainly in craters — has implications to reconstruct the climatic and geologic history of Mars. However, intra-crater dune processes on Mars are substantially affected by topographic effects on local winds, which cannot be fully resolved in Mars Circulation Models [1, 2, 3, 4]. Indeed, the Mars Science Laboratory on board of Curiosity rover landed at a crater of strong local topography (Gale crater). This crater hosts various dune fields [5] surrounding a central mountain (Aeolis Mons) that rises 5.5 km high from the crater floor. Although data on wind direction and speed are available for Curiosity landing site [1, 2], further modelling is needed to elucidate regional patterns of Aeolian shear stress driving the formation of dune fields over Gale crater. Here we conduct such a modelling by means of CFD as summarized below.
Numerical experiments
We compute the average turbulent surface wind shear stress over Gale crater’s digital terrain model (50 m resolution in horizontal direction) from the High Resolution Stereo Camera of Mars Express. The fluid (Martian air) is regarded as incompressible and Newtonian, and FLUENT Inc. commercial package is used to solve the Reynolds-averaged Navier-Stokes equations with the SST-k-ω turbulence model and hydrostatic pressure. For every incident wind direction considered, a logarithmic wind profile is imposed over the pre-crater surface level at the inlet, assuming a surface roughness of 7 mm and wind shear velocity values in the range from 0.2 m/s to 1.0 m/s (comparable to today’s winds on Mars).
Results and discussion
Fig. 1 displays results obtained by assuming south and east winds, each with wind shear velocity 1.0 m/s or upwind shear stress 0.019 Pa (resolution of CFD simulation is 250 m). Average values of shear stress predicted for Curiosity landing area are comparable to the minimal threshold shear stress for sustained transport predicted from models (0.3 − 1.0 cPa; see Ref. [7]). Our simulations could thus help to constrain upwind values of shear stress from wind observations in the landing site. However, we also identify areas of strong backward flow and regions to the east and west of Aeolis Moons in which the shear stress is below threshold without regard of the incident wind direction. Such regions may play an important role for sand deposition. Therefore, we are downscaling the simulations to 50 m resolution in dune field areas [5] to get a better understanding of local topographic effects. Furthermore, we extended a morphodynamic dune model to include an interface to CFD programs and study the dune effect [8] on the shear stress. This effect can be resolved by adding the shear stress perturbation (obtained through an analytical model, as reviewed in Ref. [7]) to the forcing, local shear stress predicted with CFD. Our coupled simulations will be helpful, for instance, as a means for testing hypotheses on (upwind) regional flow directionality and the history of sediment transport in Gale crater, as well as in other areas where strong local topography could affect dune field processes.
Acknowledgements
We thank the German Research Foundation (DFG) for funding through the Heisenberg Programme and grant 403438892.
References
[1] Pia-Garcia, J., Rafkin, S. C. R., Kahre, M., Gomez-Elvira, J., Hamilton, V. E., Navarro, S., Torres, J., Marín, M. and Vasavada, A. R. The meteorology of Gale crater as determined from rover environmental monitoring station observations and numerical modeling. Part I: Comparison of model simulations with observations. Icarus 280, 103-113 (2016).
[2] Newman, C. E., Gómez-Elvira, J., Marin, M., Navarro, S., Torres, J., Richardson, M. I., Battalio, M., Guzewich, S. D., Sullivan, R., de la Torre, M., Vasavada, A. R. and Bridges, N. T. Winds measured by the Rover Environmental Monitoring Station (REMS) during the Mars Science Laboratory (MSL) rover’s Bagnold Dunes Campaign and comparison with numerical modeling using MarsWRF. Icarus 291, 203-241 (2017).
[3] Baker, M. M., Lapotre, M. G. A., Minitti, M. E., Newman, C. E., Sullivan, R., Weitz, C. M., Rubin, D. M., Vasavada, A. R., Bridges, N. T. and Lewis, K. W. The Bagnold Dunes in southern summer: Active sediment transport on Mars observed by the Curiosity rover. Geophysical Research Letters, 45, 8853-8863 (2018).
[4] Love R., Jackson, D. W. T., Michaels, T., Smyth, T. A. G., Avouac, J.-P., Cooper, A.: From Macro- to Microscale: A combined modelling approach for near-surface wind flow on Mars at sub-dune length-scales. PLoS ONE 17(11), e0276547 (2022).
[5] Hobbs, S. W., Paull, D. J. and Bourke, M. C. Aeolian processes and dune morphology in Gale Crater. Icarus 210, 102-115 (2010).
[6] Launder, B. E. and Spalding, D. B. The numerical computation of turbulent flows. Computer methods in applied mechanics and engineering, 3(2):269-289 (1974).
[7] Parteli, E. J. R. Physics and modelling of wind-blown sand landscapes. In: Shroder, J. J. F. (Ed.), Treatise on Geomorphology (Second Edition), vol. 8. Elsevier, Academic Press, Chapter 7.03, pp. 20-52 (2022).
[8] Jackson, D., Bourke, M. and Smyth, T. The dune effect on sand-transporting winds on Mars. Nature Communications 6, 8796 (2015)
Figure 1: Shear stress (τ ) over Gale crater for a south (top) and east (bottom) incident wind (shear velocity 1.0 m/s). Shown are the shear stress components in the west-east (on the left) and south-north (on the right) directions. Each subfigure has dimensions 220 x 220 km, and the rectangle indicates Curiosity’s landing area.
How to cite: Jankowiak, J., Seybold, H., Tirsch, D., and Parteli, E.: Wind shear stress over Gale Crater, Mars, from CFD simulations , Europlanet Science Congress 2024, Berlin, Germany, 8–13 Sep 2024, EPSC2024-598, https://doi.org/10.5194/epsc2024-598, 2024.
Introduction: The current NASA's Mars 2020 mission is exploring the Jezero crater that was once filled with water. Since it is widely acknowledged that access to liquid water is essential for life, studying the fluvial activity in Jezero can aid in the crater’s habitability assessment. We examined ten water-related processes using water and sediment transport models by [3]: 1) the western inlet valley carving, 2) the northern inlet valley carving, 3) crater flooding by only northern inlet, 4) by both northern and western inlets, 5) erosion of the western rim by the western inlet, 6) erosion of the eastern rim due to the outlet, 7) water outflow from the crater, 8) outlet valley carving, 9) western delta deposition, 10) northern delta deposition. The goal of our study was to calculate the minimum timescales for each event and estimate the minimum volume of water provided/released during each event. Relative comparison of timescales and of water amount which we calculated based on new geomorphological observations introduces a deeper understanding of the water history in Jezero.
Data: Measurements of channel sizes, valleys, deltas, eroded rims, and outflowed water volumes were based on Mars 2020 Science Investigation CTX DEM Mosaic [5] and HRSC Mars Chart (HMC) DTM and corresponding orthomosaics [2].
Geomorphological observations: The northern inlet was most likely involved in the crater flooding because it has terraces at the same height as the breaching terraces in the eastern rim (breaching happened in 3 phases, as shown in [7]). The western inlet, in contrast, has no terraces at the breaching heights. This implies that either it was not involved in crater flooding, or its terraces were eroded.
Mapping: Both deltas were mapped using three potential extents: minimum, medium, and maximum. Valleys were mapped based on two morphological features: 1) initial valley, borders of which are not visible on HMC ortho-mosaics and could only be recognized on slope and profile curvature rasters, calculated from HMC DTM; 2) last incision valley, which was mapped on HMC ortho-rectified image mosaic.
Measurements: Dimensions of the channels were derived from longitudinal and cross-sectional profiles on CTX DTM. Water volume to fill the crater before breaching and the amount of sediments, transported from valleys and deposited in deltas were estimated using ArcGIS Tools „Surface Volume“ and „CutFill“.
Methodology: Flow discharge and sediment transport models [3] are used to calculate water and sediment transport timescales under constant bank-full discharge when most of erosion occurs. The models do not include the climate and non-bank-full conditions to constrain minimum timescales. Main input parameters include: Median Grain Size, Channel depth, width and slope, Sediment porosity, Shields criterion for incipient motion, Sediment density, Martian gravity, and Water density. Data from the Perseverance rover [1] were taken for the Median Grain Size estimation. Sediment porosity, Sediment density, and Shields criterion for incipient motion were taken based on previous research [3], [4], [6]. Several scenarios were modeled with varying values of input parameters in the most expectable ranges. Eastern and western rim breechings were modelled both in a catastrophic scenario and in a long-term erosion under constant flow scenario.
Results: A comparison of the timescales of the last incised valleys carving and delta depositions showed that deltas were deposited during the last incision of the corresponding valleys. For the northern delta, the medium extent is the most probable; for the western delta, the maximum extent is the most probable.
Most modelled cases, in both long-term and catastrophic scenarios of the rim breaching, showed that the outlet valley carving lasted longer than the eastern rim erosion. Therefore, Jezero was an open-basin lake after breaching.
The eastern rim erosion and water outflow during breaching showed different results depending on the scenario. In the long-term scenario, the eastern rim erosion lasted longer than the outflow of water which was stored in the crater before breaching. That means, that this amount of water (236 km3) was not enough to carve the breach. In the catastrophic scenario there are overlapping timescales, therefore, the eastern rim breaching, and water outflow could happen simultaneously.
Multiplying the timescales by corresponding discharges allows us to calculate the minimum water volume provided/released during each event. Dividing the minimum water volume by the volume of the crater before breaching (446 km3) shows that the northern inlet as well as the western inlet could alone flood the crater (last column in Table 1).
A comparison of the minimum amount of water discharged after the breach (236 km3) with the amount of water needed to carve the whole outlet valley (1000 – 14800 km3, Table 1) confirms that Jezero must have been an open-basin lake after the breaching.
Table 1. Minimum water volume provided/released during the carving of the valleys. Timescales presented for the total valley carving (initial valley and last incised valley together).
|
Timescale, Earth years |
Discharge, km3/day |
Minimum volume of provided/released water, km3 |
How many times Jezero could be filled before breaching (basin volume = 446 km3) |
Northern Valley Total |
70 – 632 |
~0.2 |
4600 – 42000 |
10 – 94 |
Western Valley Total |
505 – 3792 |
~0.2 |
33500 – 253000 |
75 – 567 |
Outlet Valley Total |
1.4 – 21.2 |
~1.9 |
1000 – 14800 |
– |
References:
[1] Farley K., and Stack K. (February 15, 2023). Mars 2020 reports, Volume 2. https://mars.nasa.gov/internal_resources/1656/
[2] Gwinner, K. et al. (2016). The High Resolution Stereo Camera (HRSC) of Mars Express and its approach to science analysis and mapping for Mars and its satellites. Planetary and Space Science, 126, 93–138. https://doi.org/10.1016/j.pss.2016.02.014
[3] Kleinhans, M. G. (2005). Flow discharge and sediment transport models for estimating a minimum timescale of hydrological activity and channel and delta formation on Mars. Journal of Geophysical Research: Planets, 110(12), 1–23. https://doi.org/10.1029/2005JE002521
[4] Kleinhans, M. G., van de Kasteele, H. E., & Hauber, E. (2010). Palaeoflow reconstruction from fan delta morphology on Mars. Earth and Planetary Science Letters, 294(3–4), 378–392. https://doi.org/10.1016/j.epsl.2009.11.025
[5] Malin, M. C. et al. (2007). Context Camera Investigation on board the Mars Reconnaissance Orbiter. Journal of Geophysical Research: Planets, 112(5). https://doi.org/10.1029/2006JE002808
[6] Roda, M. et al. (2014). Catastrophic ice lake collapse in Aram Chaos, Mars. Icarus, 236, 104–121. https://doi.org/10.1016/j.icarus.2014.03.023
[7] Salese, F. et al. (2020). Estimated Minimum Life Span of the Jezero Fluvial Delta (Mars). Astrobiology, 20(8), 977–993. https://doi.org/10.1089/ast.2020.2228
How to cite: Ovchinnikova, A., Jaumann, R., Walter, S. H. G., Gross, C., Zuschneid, W., and Postberg, F.: Water and Sediment Transport Processes in Jezero Crater, Europlanet Science Congress 2024, Berlin, Germany, 8–13 Sep 2024, EPSC2024-748, https://doi.org/10.5194/epsc2024-748, 2024.
Introduction and objectives: Despite currently being an arid planet, Mars is believed to once have had a milder climate, with a dense atmosphere capable of supporting a hydrological cycle. The sedimentary deposits putatively of deltaic origins found across all Martian surface, are good indicators of water distribution and can provide valid information to this climatic evolution [1, 10].
The Mars 2020 Perseverance rover has collected samples from the sedimentary deposit and has moved on to the Neretva Vallis. In this same area, sediments containing hydrated silica were previously identified [2]. There are also stratigraphic and morphological evidences that allow defining different phases of progradation and transgression, as well as a phase of erosion subsequent to the deposition of the sedimentary body [3]. This set of evidence suggests that this deposit was formed by fluvio-deltaic processes [4] at the end of the Noachian and beginning of the Hesperian [5].
With this work, we seek to better understand the different evolutionary phases of this fluvio-deltaic system, proceeding with a mass balance analysis to evaluate deposition and exhumation processes [6]. These phases can provide key insights into better understanding the possibility of the existence of a large body of water in the northern hemisphere [7], particularly through a comparison with the knickpoints proposed by Duran et al. (2019) [8].
Data and methods: In order to cover the entire area under study, 21 digital terrain models and their respective orthoimages were created. These data were produced using images from the Context Camera (CTX), processed using ISIS (Integrated Software for Imagers and Spectrometers) and NASA Ames Stereo Pipeline.
Vaz et al. (2020) [10] identified the existence of two different groups of fan-shaped deposits on the Martian surface. To do so, a mass balance analysis was conducted between the material removed from the valley and the material deposited at its mouth. Volumes were estimated from digital terrain models, using the methodologies described in Vaz et al. (2020) [10].
Preliminary results: After mapping the valley, deposits, and crater (basin) (Fig. 1), volume calculations were performed, and several discontinuities on the valley longitudinal profile were identified. We associate these discontinuities with different phases of valley incision.
Figure 1 - Mapped valley (blue), thalweg line (light blue), deposits (yellow) and crater (red)
Initially, only two possible scenarios were considered: a) the entire valley (Neretva Vallis) and the visible deposited material of the western deposit; and b) only the portion of the valley closest to the crater and the deposit inside the crater (Fig. 2). Considering a deposit porosity of 0.3, a valley porosity of 0.25 [9], and an erosion rate of 2.7 nm/year, we obtain: scenario a) the volume of material removed from the valley is about ten times greater than the volume of deposited material; scenario b) the material removed from the section of the valley closest to the crater is not sufficient to fill the adjacent deposit.
Figure 2 - above) Color grading of the deposit’s depth (from blue to red). Below) Color grading of the first valley section depth (from blue to yellow).
Discussion and work to be done: Currently, from the preliminary results, the mass deficit calculated for the maximum extent of the valley stands out. The material deposited in the fan only corresponds to approximately 8% of the material that was eroded to form the valley. To further evaluate these values, different parameters will be tested, namely porosities and erosion rates. The original extent of the deposit, which goes beyond the initially mapped area in this work (e.g., Kodiac Butte), will also be studied. Subsequently, information on the different known knickpoints [8] will be incorporated to try to explain the different valley sections. One other objective is to try to correlate these sections with the different depositional phases captured by the Perseverance rover in situ [11].
Acknowledgments: CITEUC (UID/Multi/00611/2021&POCI-01-0145-FEDER-006922), FCT (2021.05116.BD & CEECIND/02981/2017) and the Laboratory of advanced computation of the University of Coimbra for the computing resources.
References:
[1] Di Achille, G., & Hynek, B. M. (2010a). Deltas and valley networks on Mars: Implications for a global hydrosphere. In Lakes on Mars (pp. 223–248). Elsevier.
[2] Pan, L., Carter, J., Quantin-Nataf, C., Pineau, M., Chauviré, B., Mangold, N., Le Deit, L., ... (2021). Voluminous silica precipitated from martian waters during late-stage aqueous alteration. Planetary Science Journal, 2(2).
[3 Goudge, T. A., Mohrig, D., Cardenas, B. T., Hughes, C. M., & Fassett, C. I. (2018). Stratigraphy and paleohydrology of delta channel deposits, Jezero crater, Mars. Icarus, 301, 58–75.
[4 Fassett, C. I., & Head, J. W. (2005). Fluvial sedimentary deposits on Mars: Ancient deltas in a crater lake in the Nili Fossae region. Geophysical Research Letters, 32(14), 1–5.
[5] Mangold N., & Quantin-Nataf C. (2021). Perseverance rover reveals an ancient delta-lake system and flood deposits at Jezero crater, Mars. Science.
[6] Quantin-Nataf, C., Holm-Alwmark, S., Lasue, J., Calef, F. J., … (2021). The Complex Exhumation History of Jezero Crater Floor Unit. 52nd Lunar and Planetary Science Conference.
[7] Di Achille, G., & Hynek, B. M. (2010b). Ancient ocean on Mars supported by global distribution of deltas and valleys. Nature Geoscience, 3(7), 459–463.
[8] Duran, S., Coulthard, J., & Baynes, E. R. C. (2019). Knickpoints in Martian channels indicate past ocean levels. Scientific Reports, 9(1).
[9] Macke, R.J., Kiefer, W.S., Britt, D.T., Irving, A.J., Consolmagno, G.J., 2011. Densities, porosities and magnetic susceptibilities of meteoritic lunar samples: early re- sults. In: 42nd Lunar and Planetary Science Conference, LPI Contribution No. 1608. The Woodlands, Texas, p. 1986.
[10] Vaz, D. A., Di Achille, G., Hynek, B. M., Nelson, W., & Williams, R. M. E. (2020). Martian fan deposits: Insights on depositional processes and origin from mass balance survey. Earth and Planetary Science Letters, 533.
[11] Mangold, N., Caravaca, G., Gupta, S., Williams, R. M., Dromart, G., Gasnault, O., ... & Wiens, R. C. (2024). Architecture of Fluvial and Deltaic Deposits Exposed Along the Eastern Edge of the Western Fan of Jezero Crater, Mars. Journal of Geophysical Research: Planets, 129(3), e2023JE008204.
How to cite: Silva, D. C. A., Vaz, D., Le Deit, L., and Di Achille, G.: Jezero crater fluvio-deltaic system: insights on its geological evolution through a sedimentary mass balance modelling, Europlanet Science Congress 2024, Berlin, Germany, 8–13 Sep 2024, EPSC2024-893, https://doi.org/10.5194/epsc2024-893, 2024.
INTRODUCTION
Deltas on Mars are crucial markers for reconstructing the planet's past climatic and hydrological conditions. The detailed study of Martian deltas, particularly through the interpretation of high-resolution imaging and geomorphological mapping, reveals insights into the environmental changes the planet has undergone. This study investigates stepped-fan deposits on Mars. The research utilized remote sensing techniques and landscape analysis to produce geomorphological maps of two key sites: Picardi crater in Terra Sirenum and Dukhan crater in Xanthe Terra (Fig. 1). These sites showcase extremely well-preserved stepped-fan deposits, possibly have formed during groundwater sapping events in the early Amazonian [1], [2].
Figure 1: A) Location of stepped-fan deposits in Picardi (Terra Sirenum) and Dukhan Crater (Xanthe Terra). B) Stepped-fan in Picardi crater. C) Stepped-fan deposits in Dukhan crater.
METHODS
We used data from NASA's MRO mission (HiRISE and CTX imagery and DEMs) to produced detailed geomorphological maps of the study sites. The maps were generated using a combination of two geomorphological keys. The planetary symbolization key by the Federal Geographic Data Committee of the United States (U.S.G.S., 2006) lacks the representation of small-scale features like alluvial fans, deltas, and various surface textures observed at the sites. Therefore, a synergistic mapping approach was adopted by also integrating the symbology of the cartographic standard proposed by the Italian Geological Survey and the Italian Association of Physical Geography and Geomorphology (Gruppo Nazionale Geografia Fisica e Geomorfologia CNR, 1986).
RESULTS
Picardi crater showcases an exceptionally well-developed stepped-fan deposit [3], [4]. A multitude of geomorphological features and deposits, related to impact cratering, paleochannel activity, aeolian erosion and deposition, mass wasting and tectonism, have been identified . Most intriguing are the stepped, fan-shaped deposits in the southeastern part of Picardi crater, which have been further subdivided into individual geomorphic units, each differentiated by their specific morphometrical and sedimentological characteristics, and potential origin. The fan is connected to a small, steep-walled amphitheater-headed channel. Seven distinct fan units, originating from a single amphitheater-headed valley, were deposited radially outward from the apex (Fig. 2).
Figure 2: Detailed HiRISE images of the Picardi crater fan deposit. A) Fan Unit 1. Wrinkle ridge and smooth, sparsely cratered sediments of Fan Unit 1 distal to the main fan-geological units. B) Fan Unit 5. Finger-like extensions at the topographically low, distal part of the main fan. C) Secondary, isolated fan deposit. D) Upper part of the fan deposits with Fan Units 6 and 7 covering the highly eroded, semicircular Fan Unit 4. North is up in all panels.
Dukhan crater exhibits a diverse array of features, including plains, plateaus, tectonic structures, impact craters, and various depositional features such as fluvial, gravitational, and aeolian deposits (see figure 1c and 3). The stepped-fan deposits in Dukhan crater consist of distinct units with varied characteristics. Fan Unit 1, the most distal, has a wedge-like shape with sparse cratering and large boulders on its flat top. Fan Unit 2 is partially covered by Unit 4 and displays a slightly higher albedo with erosion on its distal side. Fan Unit 3, with a smooth surface and minor disruptions, forms the bulk of the fan. Unit 4, at the uppermost part, features asymmetrical geometry and erosion on its eastern side. The fan is connected to an amphitheater-headed inlet valley, aligned with a wrinkle ridge system on the highland plateau, suggesting underlying structural control. Along the crater walls, multiple channel-like features indicate the presence of alluvial deposits and paleochannels, gradually transitioning from rugged terrain to linear paths with changes in albedo.
Figure 3: Detailed HiRISE images of the Picardi crater deposit. A) Fan Unit 1. Wrinkle ridge and smooth, sparsely cratered sediments of Fan Unit 1 distal to the main fan-geological units. B) Fan Unit 5. Finger-like extensions at the topographically low, distal part of the main fan. C) Secondary, isolated fan deposit. D) Upper part of the fan deposits with Fan Units 6 and 7 covering the highly eroded, semicircular Fan Unit 4. North is up in all panels.
DISCUSSON
The stepped-fan deposits in Picardi crater exhibit a complex evolution, transitioning from deltaic depositional in the lower fan to alluvial dominance in the upper fan. In the middle section Unit 4 (fig. 2d), characteristics of glacial activity were recognised. The formation mechanisms of these units suggest a dynamic interplay of environmental factors, including shifts in climate and hydrological activity as well as glacial activity.
In Dukhan crater, the lowermost unit displays large boulders on its surface with diameters of >1 m and displays a massive sedimentary unit. It likely formed as a result of a landslide, and initiated the formation of the amphitheater-headed channel as a result of groundwater sapping. The upper units, resembling conical, layered deposits, have characteristics of both alluvial fans and deltaic deposits, making their differentiation challenging. While they share similarities with deltaic deposits found in other Martian regions, their lack of typical features suggests an alternative formation process, possibly through alluvial mechanisms driven by groundwater aquifers.
CONCLUSIONS
The study reveals a more complex formation history for Martian stepped-fans than previously recognized. Both Picardi and Dukhan craters show evidence of multiple processes, including deltaic, alluvial, and glacial and mass wasting processes. These findings enhance our understanding of Mars' climatic and hydrological evolution, indicating shifts from water-rich to glacial and arid conditions over time.
REFERENCES
[1] E. Hauber et al., ‘Asynchronous formation of Hesperian and Amazonian-aged deltas on Mars and implications for climate’, Journal of Geophysical Research: Planets, vol. 118, no. 7, pp. 1529–1544, 2013, doi: 10.1002/jgre.20107.
[2] E. Hauber et al., ‘Old or not so old: That is the question for deltas and fans in Xanthe Terra, mars’, presented at the Third Conference on Early Mars, 2012.
[3] E. R. Kraal, M. van Dijk, G. Postma, and M. G. Kleinhans, ‘Martian stepped-delta formation by rapid water release’, Nature, vol. 451, no. 7181, pp. 973–976, Feb. 2008, doi: 10.1038/nature06615.
[4] G. G. Ori, L. Marinangeli, and A. Baliva, ‘Terraces and Gilbert-type deltas in crater lakes in Ismenius Lacus and Memnonia (Mars)’, Journal of Geophysical Research: Planets, vol. 105, no. E7, pp. 17629–17641, 2000, doi: 10.1029/1999JE001219.
How to cite: Sassenroth, C., Hauber, E., Salvatore, M. C., and Baroni, C.: Spatiotemporal development of two stepped fans in Xanthe Terra and Terra Sirenum, Mars, Europlanet Science Congress 2024, Berlin, Germany, 8–13 Sep 2024, EPSC2024-1355, https://doi.org/10.5194/epsc2024-1355, 2024.
Please decide on your access
Please use the buttons below to download the supplementary material or to visit the external website where the presentation is linked. Regarding the external link, please note that Copernicus Meetings cannot accept any liability for the content and the website you will visit.
Forward to presentation link
You are going to open an external link to the presentation as indicated by the authors. Copernicus Meetings cannot accept any liability for the content and the website you will visit.
We are sorry, but presentations are only available for users who registered for the conference. Thank you.
Introduction – On Mars, the crustal dichotomy marks the transition from ancient southern highlands to northern lowlands. Infrared datasets reveal several sites therein with extensive clay-rich deposits, further testifying for widespread aqueous conditions on early Mars [1]. Here, we investigate the deposits found in key regions along this boundary (Fig. 1) [2,3]. Clay deposits are perfect “windows” to search for signs of life on the planet, as clays are known to accumulate and preserve organic compounds [4]. Infrared spectroscopy is a powerful tool to constrain the surface composition as near-infrared spectra show diagnostic bands. Knowing the exact positions of absorption band centers is therefore essential to ascertain possible species, and search for changes in clay mineralogies associated with differences in formation and alteration conditions. We examine hyperspectral data to better characterize the near-infrared signatures of clay deposits, and also verify for eventual mixing with carbonates.
Figure 1 - Mars with colorized topography (MOLA), and regions included in this survey.
Data & Methods – Spectral signatures of clays are obtained from infrared data gathered by the CRISM instrument [5]. We used ~100 CRISM cubes acquired in the infrared range (1–4 µm), targeting regions selected along the dichotomy. They were pre-processed with the CAT ENVI toolkit for basic atmospheric and photometric corrections. Corrected cubes were also denoised to reduce noise and residual atmospheric contributions, and finally emphasize mineralogical absorptions. We calculated band depths at 1.9 and 2.3 µm [6] to select pixels with strong paired absorptions and outline the clays to define regions of interest (ROIs) for each cube.
Clay Diversity? – We retrieved the band centers for all pixels composing the ROIs within the absorptions of interest. Band centers obtained for most outcrops generally correlate with ferrosaponites or vermiculites, with average values being centered around 1.410, 2.305, and 2.397 µm, notably in Oxia Planum [2,7]. Some exceptions are observed, like in Mawrth Vallis where absorptions are slightly shifted to 1.42, 2.29 and 2.40 µm, rather consistent with nontronites. The exact position therein depends on the relative abundance of iron and magnesium in the clay structure, or even the oxidation state of iron [8]. Overall, these “intermediate” clays correspond to Fe-rich species, particularly ferrous smectites with a trioctahedral composition. Conversely, nontronites are rather ferric smectites with a dioctahedral composition. Interestingly, ferric smectites may form from oxidation of the ferrous smectites. The reducing atmosphere of early Mars would favor the formation of ferrous smectites, while their subsequent oxidation would explain the presence of ferric smectites nowadays [e.g., 9,10].
Possible Carbonates? – All CRISM cubes analyzed display an additional, shallow absorption centered near 2.5 µm, which is detected across all clay-bearing outcrops. Such an absorption could indicate the presence of carbonates intermixed with clays [e.g., 2,8,11]. Carbonates are usually best identified by paired absorptions at 2.3 and 2.5 µm, although these features are masked by the clays. An absorption near 2.53 µm would be consistent with Fe-rich carbonates (siderites), whereas an absorption at shorter wavelengths is often associated with Mg-rich carbonates (magnesites) [12]. Clay-bearing outcrops mainly present an absorption centered at 2.53 µm. Interestingly, the McLaughlin crater also bears outcrops with an absorption at 2.50 µm associated with Mg-rich clays (saponites).
We also searched for a specific pattern in the 3–4 µm range, where a broad peak is expected between deep absorptions occurring near 3.4–3.5 µm and 3.9 µm. We computed the band depth near 3.9 µm [11,12] to display the presence of carbonates intermixed with clays (Fig. 2). A clear absorption near 3.4–3.5 µm is generally missing in our spectra, likely due to deep water absorption near 3 µm induced by clays. This prevents a definitive characterization of carbonates. Nonetheless, the coprecipitation of clays and carbonates throughout the outcrops further strengthens the exobiological potential of the selected regions, where biosignatures might still be preserved.
Figure 2 - (A) False-color “RGB” composites. (B) Fe,Mg-rich clay deposits combining absorptions at 1.4, 1.9, 2.3, and 2.4 µm (also near 2.5 µm). (bottom) “BD3900” index outlines the drop of reflectance near 3.9 µm, and may indicate the presence of carbonates with the clays.
Conclusions – We follow-up recent investigations where we perform spectral surveys on clay deposits found in key regions along the Martian crustal dichotomy [2,3], and compare them with carefully selected terrestrial analogs. By doing that, we retrieved the exact positions of absorption band centers, and searched for possible variations therein. Variations observed between the targeted clay deposits suggest subtle changes in the iron and magnesium content, and also the oxidation state of iron. Mineralogical similarities observed in most regions suggest that clay deposits (ferrosaponites or vermiculites) may share a common weathering history. Conversely, nontronites extensively detected near Mawrth Vallis and surroundings indicate a different formation and alteration setting. Nonetheless, the region may have shared a common aqueous history with other deposits found elsewhere on the planet, and then diverged to form more leached or oxidized clays with Al,Fe-rich compositions [13].
The presence of additional absorptions near 2.5 µm and 3.9 µm typical of carbonates, may indicate their presence within clay deposits. This testifies for a widespread distribution of carbonates on Mars, more ubiquitous than previously thought.
Funding. This work is supported by the Italian Space Agency (ASI) [Grant ASI-INAF n. 2023-3-HH.0].
References. [1] Carter et al. (2013) JGR. [2] Brossier et al. (2022) Icarus. [3] Brossier et al. (2023) PSJ. [4] Vago et al. (2017) Astrobiology. [5] Murchie et al. (2007) JGR. [6] Viviano-Beck et al. (2014) JGR. [7] Mandon et al. (2021) Astrobiology. [8] Michalski et al. (2015) EPSL. [9] Chemtob et al. (2015) JGR. [10] Chemtob et al. (2017) JGR. [11] Bultel et al. (2019) JGR. [12] Ehlmann et al. (2008) Science. [13] Carter et al. (2015) LPSC Abstracts.
How to cite: Brossier, J., Altieri, F., De Sanctis, M. C., Frigeri, A., Ferrari, M., De Angelis, S., Bruschini, E., and Apuzzo, A.: Clay - Carbonate Assemblages along the Martian Crustal Dichotomy: A Key to Assess Aqueous Conditions on Early Mars, Europlanet Science Congress 2024, Berlin, Germany, 8–13 Sep 2024, EPSC2024-271, https://doi.org/10.5194/epsc2024-271, 2024.
Deltas record fluvial and sedimentary activities on the early Mars. Yet, quantitative hydrologic and sedimentary processes related with their formation need further investigation. Here we quantitatively analysed the fluvial and sedimentary process of seven deltas exhibiting diverse morphologies across different Martian locations. Identification of Martian rivers from valleys formed the basis of our analysis, with estimates made for paleo discharge, sediment transport rates, timescales and requisite water for deltas construction. We considered various water supply processes, and classified the seven deltas into three types based on geological contexts of their supplying valleys and water source. We suppose that the delta formation potentially associated with various scenarios, including retreating erosion, groundwater sapping, ice melting, precipitation, impact events and variations in Martian obliquity, unveiling that a warm climate is not a fundamental requirement for the formation. Based on mass balances, the continuous formation timescales of these deltas range from days to thousands of Martian years. Considering the intermittency of water supply and flow dynamics, these timescales can extend to hundreds of millions of years. These findings allow for quantitatively analysing paleo-sedimentary processes that may preserve geological and climatic cues.
How to cite: Luo, Y.: Constraints on paleo hydrological activities on Mars derived from delta formation, Europlanet Science Congress 2024, Berlin, Germany, 8–13 Sep 2024, EPSC2024-841, https://doi.org/10.5194/epsc2024-841, 2024.
Introduction
Today, Mars’ surface is defined as a cold hyper-arid desert, but geomorphological and geochemical observations have shown that in the past, vast amounts of liquid water were stable at the planet’s surface (Di Achille et al. 2006, Erkeling et al. 2012, Hauber et al. 2009). With the increased coverage of high-resolution remote sensing datasets, investigations of possible remnants of fluvial processes in the Martian landscape have significantly increased during the last couple of decades. The spatiotemporal evolution of hydrological events is however not well understood (Erkeling et al. 2012). In this master thesis study, an impact crater in the region of Nilosyrtis Mensae has been mapped to identify geomorphological evidence that can increase the understanding of the spatiotemporal evolution of landforms at the dichotomy boundary on Mars.
Method & Data
The mapping was made using ArcGIS Pro version 3.1.0. with inspiration taken from the FGDC Digital Cartographic Standard for Geologic Map Symbolization (USGS, 2006) as well as the Italian cartographic standard Geomorphological Map of Italy at 1:50,000 scale by ISPRA (2021). The Context Camera [CTX] mosaic & raw images (5-6 m/px) and High Resolution Imaging Science Experiment [HiRISE] images (0.3-0.25m/px) as well as the THEMIS IR mosaic dataset of 100m/px were used to map surface features. 3D models were used in this study to support interpretations, such as the HRSC DEM product mosaic of 50m/px. By using the MarsSI application (marssi.univ-lyon1.fr) overlapping CTX raw images have been processed for HiRISE & CTX imagery to create DEM products with 12 m/px and 0.5 m/px respectively.
Geological setting
The study crater is 80+ km wide and is located at the dichotomy boundary. The crater lacks primary impact morphology and ejecta. The northeastern wall is completely degraded and opens up towards the northern plains. Moreover, the crater wall is incised by several channels of which two channels connects to adjacent craters of similar size, located east and west of the study area. The crater floor forms a palimpsest landscape of different landforms suggesting a very complex history of sedimentation, exhumation and erosion.
Result
The main result is the geomorphological map produced by this study (Fig.1). Key findings include a set of fan-shaped landforms that partially cover the crater floor. Some fans are associated with valley incisions and one of the larger fans is heavily affected by impact cratering. The fan shapes have a variety in surface texture, morphology, size and stratigraphic positions. Multiple elongated fan shapes combined with streamlined plains, alluvial deposits have been identified at the termination of the southwestern valley inlets (Fig. 2).
Figure 1. A geomorphological map of an impact crater basin in Nilosyrtis Mensae, Mars, at approximate
29°N, 72°E draped over the CTX satellite mosaic (NASA/JPL/MSSS/The Murray lab
Spatial reference: CGS Mars 2000 Sphere. Datum: Mars 2000 sphere. Map units: Degree.
Figure 2. A) 3D view of the valley inlet entering the study crater from the southeast. B) An overview of the mapped units of possible fluvial genesis in the western region of the impact crater basin. Fan shape 1 and 2 are deposited in a close spatial proximity to the channel inlet, while fan shape unit 3 is found further out, divided by a depression of possible alluvial deposition. Fan shape unit 4 covers a large part of the crater floor and does exhibit unique geomorphological features at the top of the streamlined plains marked at 5. Image credit: NASA/JPL/MSSS/The Murray lab.
Discussion & Conclusion
The diverse classification of landforms and interpreted geneses shows that the studied crater has experienced a long history of degradation, deposition by various processes and erosion since its formation. The superposition between geological units suggests a scenario where fluvial deposition have occurred episodically. Bamberg et. al., (2014) describes the geological history of the floor-fractured crater which is connected to the studied crater by a channel inlet from the northwest. The possible fluvial landforms in the studied crater suggests a close relationship to the hypothesized scenario by Bamberg et al., (2014) of a major fluvial event. Lesser fluvial activity followed, predominantly controlled by conditions in the study crater. In conclusion, the geomorphology suggests a long and complex history of fluvial events. An impact of possible lacustrine or marine conditions could not be confirmed nor refuted. The framework of the thesis did not allow for more detailed studies using spectral data. Work that includes CRISM may provide further insights into the temporal evolution in the studied crater.
References
Bamberg et.al., (2014). Floor-Fractured Craters on Mars – Observations and Origin. Planetary and Space Science, 98, 146–162. https://doi.org/10.1016/j.pss.2013.09.017
Di Achille et.al., (2006) Geological evolution of the Tyras Vallis paleolacustrine system, Mars. Journal of Geophysical Research, 111(E4), E04003-N/a.
Erkeling, et.al., (2012). Valleys, paleolakes and possible shorelines at the Libya Montes/Isidis boundary; implications for the hydrologic evolution of Mars. Icarus 219(1), 393-413.
Hargitai, (2019). Planetary Cartography and GIS. Springer.
Hauber, et.al., (2009). Sedimentary deposits in Xanthe Terra: Implications for the ancient climate on Mars. Planetary and Space Science, 57(8), 944-957
ISPRA. (2021). Geomorphological Map of Italy at 1:50,000 scale - Update and additions to the Guidelines of the Geomorphological Map of Italy at 1:50,000 scale and Geomorphological Database (Version 2.0). Volume 13, issue 1 Volume 13, Part I - The CARG Project: changes and additions to the Quaderno n. 4/19944/2022. https://www.isprambiente.gov.it/resolveuid/45c17b95e93f45cda3e1c2fa0f5e06fa
USGS. (2006). FGDC Digital Cartographic Standard for Geologic Map Symbolization (PostScript Implementation. U.S. Geological Survey Techniques and Methods 11-A2. Derived 2023-12-29 from:https://pubs.usgs.gov/tm/2006/11A02/
How to cite: Saar, E., Sassenroth, C., and Johnsson, A.: Evolution of fluvial, and possible lacustrine or marine activity in Nilosyrtis, Mars - A geomorphological & chronostratigraphic analysis., Europlanet Science Congress 2024, Berlin, Germany, 8–13 Sep 2024, EPSC2024-1077, https://doi.org/10.5194/epsc2024-1077, 2024.
Introduction
We examined an unnamed impact crater roughly centered at 27.8°N, 11.5°E, in the northeastern portion of Arabia Terra. Its uttermost relevant characteristic is the presence of multiple fan-shape deposits on its floor [1; 2; 3] exhibiting an impressive preservation of water-related features (Figure 1).
We carried out geomorphological analyses and mapping, which can provide valuable insights into the amount of water needed to generate the observed landforms, and is directly relevant for understanding the past climate and the associated geological processes. Additionally, we modeled the groundwater depth at the time of crater formation. The results of this numerical model can help us to evaluate if the observed landforms are consistent with the thermal conditions necessary for groundwater to exist at this location. These models are also used to compare the depth to the base of the cryosphere at this specific location to the planetary average at the time of crater formation. These measurements allowed us to reconstruct the different stages of aqueous activity within the crater.
Figure 1. CTX mosaic of the study area. Contour lines are displayed every 250 m using a color gradient from green (-4050 m) to orange (-2300 m). High-interest geomorphological features are mapped in blue (valleys) and brown (fan-shaped deposits).
Methods
For our geomorphological observations, we relied on imagery from the Context Camera (CTX; ∼6 m/pixel) global mosaic [4]. For a regional topographic context we used the Mars Orbiter Laser Altimeter colorized elevation model (∼460 m/pixel) [5]. Observations, mapping, and measurements were made using the QGIS software, while for age determination we used the crater size-frequency distributions (CSFD) of impact craters [6].
The CTX Digital Elevation Models (DEMs) used in this work were first downloaded as raw images from the PDS Geosciences Node. The image processing was carried out through the USGS ISIS3 [7]. The resulting point clouds were then aligned to the MOLA global topography to improve the accuracy and finally converted to DEMs.
Volumetric estimations were carried out using the 3D point cloud and mesh processing CloudCompare (CC) software (cloudcompare.org) [8]. In the CC environment, through the 2.5D Volume Calculation Tool, it is possible to estimate volumes between a point cloud and an arbitrary plane, thus efficiently simulating the given case scenario of water volumes inside an impact crater.
We used global thermal evolution models [9] to estimate the groundwater depth at the time of the crater formation by comparing the local subsurface temperature with the melting temperature of water ice. Our models employ the most recent constraints on the interior structure of Mars from InSight seismic data [10]. The crustal thickness in our models is constant in time but varies locally according to crustal thickness maps derived from gravity and topography data and anchored by seismic measurements at InSight landing site [11]. We used several crustal thickness models [11] to test the effects of crustal thickness variations on the depth of groundwater.
Results
The examined crater displays numerous water-related features suggesting that during its evolution it must have experienced at least a period of intense water activity despite its relatively young age. Nevertheless, the crater is missing a connection with large-scale fluvial networks capable of surface runoff.
As the emergence of springs is connected to groundwater availability, it implies that the head of the valleys indicates the groundwater level that generates them. Accordingly, the elevation of valley head locations in the studied crater can provide us with information about groundwater supply during the period of formation. Specifically, valley heads are located close to -2900 m elevation, suggesting that this may represent the top of the groundwater table level at the time when landforms developed after the crater formation at 3.5 Ga.
Overall, from the collected evidence, we can reconstruct a timeline of key events that shaped the examined area (Figure 2). The projectile collision with the Martian surface leads to the crater excavation, reaching the depth of the groundwater table. Groundwater flows into the basin and forms the observed water-related features observed today. The exceptionally good match between geomorphological observations and the expected groundwater depth obtained through modeling suggests that the crater infill likely took place shortly after the impact when a warmer subsurface temperature and, hence, a shallower groundwater level were present. In this context, groundwater flowed from the subsurface toward the basin creating sapping valleys and filling the crater with water and fan-shaped sedimentary deposits. As the Martian subsurface cools with time, i) the water input stopped; ii) the water already present in the basin got lost in the ground and atmosphere; iii) the groundwater table reached a deeper level given the thinner, less insulating crust at the crater location, eventually arriving at a scenario comparable to that currently observable.
Figure 2. Main events of the examined area. t-1 = unperturbed pre-impact crust; t0 = impact (3.5 Ga); t1 = water sapping, valleys and fan-shaped deposits formation, the basin fills with water; t2 = the basin dries out, geomorphological features connected to water circulation are left in a subaerial environment and the groundwater level adapts to the new surficial roughness
References
[1] Irwin, R.P., et al., (2005). J. Geophys. Res. 110. [2] Salese, F., et al., (2019). JGR: Planets, 124(2), 374–395. [3] De Toffoli, B., et al., (2021). Geophys. Res. Let., 1–10. [4] Malin, M. C., et al. (2007). J. Geophys. Res 112, E05S04. [5] Zuber, M. T., et al. (1992). J. Geophys. Res. 97, 7781–7797. [6] Hartmann, W. K., & Neukum, G. (2001). Sp. Sci. Rev, 96, 165–194. [7] Becker, K. J., et al., (2007). Lun. Plan. Sci. Conf.1338, 1779. [8] Penasa, L., et al. (2021). Wiley, 3D Geo. Mod. [9] Plesa, A. C., et al., (2022). Geophys. Exp. Sol. Sys. 63, 179-230. [10] Knapmeyer-Endrun, B., et al. (2021). Science, 373(6553), 438-443. [11] Wieczorek, M. A., et al. (2022). JGR: Planets, 127, e2022JE007298.
Acknowledgement
The authors thank the European Union – NextGenerationEU and the 2023 STARS Grants@Unipd programme “HECATE” support.
How to cite: De Toffoli, B., Plesa, A.-C., Luzzi, E., and Hauber, E.: Evidence of groundwater discharge and lake formation in Arabia Terra, Europlanet Science Congress 2024, Berlin, Germany, 8–13 Sep 2024, EPSC2024-413, https://doi.org/10.5194/epsc2024-413, 2024.
- Centrum Badań Kosmicznych Polskiej Akademii Nauk (CBK PAN), Bartycka 18A, 00-716 Warszawa, Poland
Introduction
Geomorphological evidence of deglaciation was discovered on Mars at mid-latitudes [1, 2], in response to planetary obliquity changes [3]. Many craters contain evidence of subsurface ice remaining from Late Amazonian deglaciation [4]. Martian climate models predict the presence of ice accumulation in the mid-latitudes, especially on the western flanks of the Tharsis montes [5]. During the Amazonian epochs, the early Tharsis reservoirs were partly withdrawn to the surface, through outflow channels [6]. The largest of them formed between 3.7 and 3 billion years ago. There is evidence, however, that the crustal water reservoir was not exhausted after this time [7][8]. Many channels, including the largest Amazonian system Olympica – Jovis Fossae, formed at the top of the Tharsis bulge in lava flows that may be only a few hundred million years old [9]. To investigate the formation of such channel systems, we present a new geological map of Olympica-Jovis Fossae (Figure 1).
Data and methods
To map morphological and geological features, we used visible imagery from the Mars Reconnaissance Orbiter (MRO) Context Camera (CTX) with a resolution of 6 meters per pixel [10][11] and MRO High-Resolution Imaging Science Experiment (HiRISE) camera with a resolution of 25 cm per pixel [12]. To constrain the intersecting relationships between channels we used the newest DEM topography data (2021 – 2023) from the Mars Express High Resolution Stereo Camera (HRSC) with a resolution of ~20-40 meters per pixel [11][13] and optical imagery data from the ExoMars Trace Gas Orbiter, Colour and Stereo Surface Imaging System (CaSSIS) with a resolution of 4.8 meters per pixel ([14]).
Results
Our new geological map includes fluvial units (Figure 2), volcanic units, volcanic edifices and vents, glacial lineations, regional fracture systems, and impact craters. We identified 28 fluvial units that could be attributed to six classes, based on discriminating geomorphological features. Observed channel morphologies include the main channel (set of troughs and channels extending from the triple junction to Jovis Fossae), braided channels with densely interconnected channels with dendritic and sinuous geometry, terraced channels with eroded floors, step-like landforms, meandering channels, tectonically controlled channels, and outflow channels with streamlined islands. The oldest in the sequence of fluvial channels are terraced channels, representing multiple flooding events, followed by braided channels, meandering channels, outflow channels that originate at
Jovis Fossae system fissures, and tectonically controlled channels (attributed to extensional stress, subsequently developed and filled by water and lava). Investigation of the mapped units and correlation of channel formation reveal at least three bigger flooding events. We determined the relative ages and relationships between fluvial channels from cross-cutting relationships (Figure 3).
Figure 1. Geologic map of the Olympica Fossae and Jovis Fossae hydrologic system.
Figure 2. Zoom on two regions of the map. Legend: clt – collapsed lava tube, Atc – Amazonian terraced channel, Amc – Amazonian meandering channel, Abc – Amazonian braided channel, Atcc – Amazonian tectonically controlled channel.
Figure 3. Chart of cross-section relationships between 28 fluvial channels. Arrowheads indicate younger flows.
Discussion and conclusion
We present the first detailed geological map and relative dating of the Olympica Fossae and Jovis Fossae, providing a basis for understanding the recent hydrologic and volcanic activity at the top of the Tharsis dome. Our map includes 28 individual fluvial units, glacial lineations, impact craters, volcanic units, edifices, and vents, as well as geological structures. We found a series of complex cross-cutting relationships between channels. General investigation of the channel’s morphology and their correlation with volcanic edifices reveal that the Olympica Fossae and Jovis Fossae formed by repeated overflows from various fissures related to an intensive period of distributed volcanism. Olympica – Jovis Fossae shares similar fluvial morphology with other outflow channels on Mars. Since our geologic map reveals six types of channels, future detailed morphologic analysis will be crucial to better understand the general conditions for catastrophic groundwater flooding in a volcanic context. Further efforts are needed to identify the chronological succession of deposition and flows and interfaces between floods, lava flows, and airfall deposits, based on crater retention age determination.
References:
[1] Baker, D. M. H., & Head, J. W. (2015). Icarus, 260, 269–288.
[2] Head, J. W., et al. (2006). Geophysical Research Letters, 33(8).
[3] Head, J. W., et al. (2006). Earth and Planetary Science Letters, 241(3-4), 663–671.
[4] Dickson, J.L., et al. (2010). Earth and Planetary Science Letters, 294(3-4), 332–342.
[5] Shean, D. E. (2005). Journal of Geophysical Research, 110(E5).
[6] Cassanelli, J.P., Head, J.W. (2019). Planetary and Space Science 169, 45-69.
[7] Rodriguez, J. A. P., et al. (2015). Icarus, 257, 387–395.
[8] Hiatt, E., et al. (2024). Icarus, 408, 115774
[9] Keske, A.L., et al. (2015). Icarus, 245, 333-347.
[10] Fergason, R. L., et al. (2021). 51st Lunar Planet. Sci. Conf. Abstract #2020.
[11] Jaumann, R., et al. (2007). Planetary and Space Science, 55(7), 928–952.
[12] McEwen, A. S., et al. (2007). Journal of Geophysical Research, 112(E5), E05S02.
[13] Neukum, G., Jaumann, R. (2004). Eur. Space Agency Spec. Publ., ESA-SP 1240, 17–35.
[14] Thomas, N., et al. (2017). Space Sci Rev 212, 1897–1944.
How to cite: Zambrowska, A., Mège, D., and Poppe, S.: Polyphase Amazonian floods in the Olympica – Jovis Fossae channel system., Europlanet Science Congress 2024, Berlin, Germany, 8–13 Sep 2024, EPSC2024-777, https://doi.org/10.5194/epsc2024-777, 2024.
Please decide on your access
Please use the buttons below to download the supplementary material or to visit the external website where the presentation is linked. Regarding the external link, please note that Copernicus Meetings cannot accept any liability for the content and the website you will visit.
Forward to presentation link
You are going to open an external link to the presentation as indicated by the authors. Copernicus Meetings cannot accept any liability for the content and the website you will visit.
We are sorry, but presentations are only available for users who registered for the conference. Thank you.
Introduction: Glacier-like forms (GLFs) are a particular class of viscous flow formations (VFFs) that occupies the mid-latitudes of Mars [e.g., 1–4]. They appear to be concentrated around the 40–55
latitude range in both hemispheres [3,4]. Their distribution signifies changes in water-ice latitudinal stability with obliquity variations. Therefore, studying them and understanding their evolution is critical to understanding the recent glacial history of Mars and stability of ice on its surface. For this study, we focus one a GLF in Argyre (Fig. 1). In earlier updates (e.g., [5]), we showed that the glacier system displays 1) multi piedmont-like terminal lobes, 2) gullied cirque-like source regions, and 3) periglacial modification of surface materials indicative of near-surface ice. Here, we provide further updates about our mapping, which highlight the complex history of the studied region.
Methods and datasets: We carried out the morphologic investigation using images from HiRISE, CTX and CaSSIS. We created a CTX mosaic for the study region and geo-referenced it to an HRSC DTM to extract morphometric information on flow directions and influence of surrounding topography. Here we describe a number of new observations coupled with morphometric measurements where relevant.
Positive Relief Feature (PRF): The PRF is the main source region for the various VFFs observed around it (Fig. 1). It has an elevation of ~+3250 m and rises ~4250 m above the surrounding terrains to the east, and more than 6000 m above the Argyre floor to the west. It displays a wide mesa-like flat top more than 20 km across along its longest axis with slopes ranging as low as 1° up to 7° in certain areas. The mesa top appears mostly smooth with muted topography in CTX images, suggesting a recently emplaced mantling unit. In contrast, the sides of the PRF are steep (22–32°) with the NE slopes displaying the highest slopes (~32) associated with a semi-circular cirque-like alcove. Based on [6], we interpret this PRF to be an erosional remnant of the basin’s rim materials.
The lobate deposits: The lobate deposits surround the PRF and dominate the terrain from its lower flanks to regions ~10-35 km away, depending on the direction (Fig. 2). They mainly comprise a NE trending lobe, a western deposit that branches into two distinctive lobes trending NW and SW; all three whose flow directions are constrained by surrounding topography resembling Lineated Valley Fills (LVFs) [2], and finally an East-trending unit that flows more feely and displays a Lobate Debris Apron (LDA)-like morphology [2]. All flows display clear terminal ridges and flow fronts, whereas the three LVF types also display sets of quasi-parallel lineations trending along the respective flow direction. The flows show relatively low slopes (typically <5), and show clear signs of viscous deformation as evident from their flow directions around topographic obstacles and surface lineations that develop in response to these movements. The lobate flows appear smooth in CTX and CaSSIS image resolutions with very few fresh-looking impact craters. Certain outcrops that protrude above the surface of the flows display concentrations of fine-grained materials organized in ripple formations. A CaSSIS image covering the western flows acquired in Ls 157 (Southern hemisphere winter) display bright frost accumulations over the same outcrops at preferentially at the west-facing sides (Fig. 3). Notably, we observe a potential paleo drainage feature in the form of quasi-linear valley in close proximity, but not in direct contact with the (current) boundary of the NE lobe (Fig. 4). An inspection of this feature using the HRSC DTM indicates that the valley appears to originate at or beneath a region of brighter fine-grained materials at the lobate boundary, and crucially, traversing over a relatively higher elevation mound, and then extends downslope ~2 km with a near uniform width of ~150 m (Fig. 5). The area between the mound and the terminal boundary of the lobate deposits also dips in elevation. The valley can be best traced in a CaSSIS image and overall extends up to 2.7 km away from the flow boundary. We plan to better quantify the morphometry using new HiRISE DTMs to verify this stratigraphical relation, and should report about it in more detail at the meeting.
Acknowledgements: MRELM acknowledges funding from the KU internal grant (8474000336-KU-SPSG). G.A.D. is gratefully funded by an UKRI STFC Studentship.
References [1] Arfstrom, J., and Hartmann, W.K., (2005), Icarus, 174, 321–335. [2] Head. J.W. et al. (2010), EPSL, 294, 306–320. [3] Souness, C. et al. (2012). Icarus, 217, 243–255. [4] Hubbard, B. et al. (2014), Cryosphere, 8, 2047–2061. [5] El-Maarry, M.R., Diot, X. (2020), 7th Mars Polar meeting, abstract #2099. [6] Dohm, J.M. et al. (2015), Icarus, 253, 66–98.
Figure 1. [Top] Argyre basin in blended THEMIS Day IR and MOLA with a white box showing the location of the studied landform. [Bottom] 3D view compiled from an HRSC DTM with a CTX mosaic overlaid at 3x vertical exaggeration. A black arrow points to the heavily modified cirque-like accumulation region, and a yellow arrow shows the terminus of the NE lobe.
Figure 2. THEMIS Day IR mosaic of the glacial system showing the key landforms described here. White boxes show the locations of Figures 3 and 4.
Figure 3. CaSSIS RGB image acquired during Ls 157 (Southern hemisphere winter) displaying bright deposits, probably CO2 frost, accumulating over fine-grained materials that display ripple features during warmer seasons. (Image ID: MY35_010096_310_0_RGB).
Figure 4. CaSSIS image covering the NE lobe and showing the valley feature inclose proximity and appearing to flow away from the NE lobe. Note the dark band circumferential to the current lobe boundary cross-cutting the valley feature as well. (Image ID: MY35_012794_311_0_NPB).
Figure 5. CTX mosaic draped over an HRSC DTM showing the valley and the mound it traverses over. Directional lighting has been implemented to highlight the topographical relations.
How to cite: El-Maarry, M. R. and Driver, G.: Investigating the complex history of a mountain glacier in Argyre Basin, Mars: Potential for warm-based glaciation?, Europlanet Science Congress 2024, Berlin, Germany, 8–13 Sep 2024, EPSC2024-758, https://doi.org/10.5194/epsc2024-758, 2024.
The ChemCam instrument onboard the Mars Science Laboratory has detected elevated values of Lithium (Li) in Si-Al-filled fractures throughout the sedimentary layers of the crater Gale. Previous studies have associated the abundance of Li in calcium sulphate veins with the degree of dehydration of diagenetic fluids [1], but the final reservoir of Li in the last evaporation stage remains unknown. Since elevated Li abundance in secondary minerals provides insights into the extension and intensity of Martian aqueous processes [2, 3], we evaluated the geochemical significance and possible mechanisms of Li incorporation into their minerals.
The Li concentration of the rock as a whole along the stratigraphic sequence (Figure 1) shows average values similar to Earth's Middle Ocean Ridge basalts (MORB ~ 6,5 ppm) [4]. The variations in Li concentration with respect to the MORB protolith exhibit a range of ± 6,5 ppm at the majority of observation points, although there are two noteworthy exceptions. The first deviation corresponds to the Bradbury group at the crater base, where the observed Li abundance deviates by up to 120 ppm. This pattern follows a decreasing trend between members, with the Li content stabilising at values closer to MORB. The second deviation corresponds to the Glasgow member, which shows a high Li abundance in fracture-fill sediment, with values ranging from 45 to 125 ppm, with an average of 72 ppm (Figure 2). In this latter case, Li is explicitly associated with Silica-Alumina veins that cut through the fractured basalt mudstone and separate areas of veins rich in Mn/Fe minerals. Preliminary calculations based on the composition of Al2O3 and SiO2 oxides indicate that the stoichiometry of double Al-silicates such as kaolinite or halloysite alone does not fit the observed composition of the veins. This suggests that a non-stoichiometric mixture of amorphous silica together with various aluminosilicates capable of incorporating Li, K and Mg into their structure forms the observed veins.
Here, we analyse the incorporation of Li into amorphous or partially crystallised materials derived from circulating silica-aluminium-rich solutions of basaltic weathering. These solutions have the potential to precipitate both crystalline aluminosilicates with structural Li and Al-Si hydroxide gels, which are capable of intercalating Li into their amorphous network through reverse weathering. We included in the database the possible Al-Si phases formed in the final stages of Li evaporation brines, to determine their supersaturation states. We used the LiMars code, a set of scripts based on PHREEQC software [5], which allows the geochemical modelling of Li dissolution processes from primary minerals and the kinetic precipitation of crystalline and amorphous aluminosilicate phases. The incorporation of Li into these secondary phases is an adsorption or ion exchange mechanism. The geochemical scenario involves two solutions with opposing pH values, mixing by diffusion in an evaporative context. Depending on the pH conditions, the volcanic glass may undergo alteration into a variety of mineral assemblages. This may occur under alkaline conditions, where it may transform into Fe and Mn oxides, or during evolution to more acidic conditions, where it may evolve into hydrated volcanic glass, amorphous silica, kaolin minerals or Li-bearing Al-Si minerals. Experiments conducted with Al-Si gels formed in brines [6] showed that the highest adsorption capacity is observed at pH values of 7 - 8, which is close to the zero-loading point, and the adsorption capacity decreases at both alkaline and acidic values. This pH-dependent behaviour indicated that the neutral medium, where amorphous gels and clays precipitate, is the most favourable for Li adsorption. We hypothesise that the geochemical significance of these Al-Si veins in the Glasgow member could represent the latest stages of the evolution towards equilibrium of diagenetic fluids in Gale.
Figure 1. Individual ChemCam observation points plotted against elevation relative to the Martian datum for Li (ppm). The colour indicates the stratigraphic member where the points were collected. All observation points with a total sum of oxides less than 90 wt.% and those located more than 3.5 m from the ChemCam instrument were discarded. Data from Planetary Data System (PDS) Geoscience Node [7].
Figure 2. High-resolution Remote Micro-Imager (RMI) context picture of the chemical analyses performed by LIBS. Targets: Glenmorrison, Glen_cannich and Glen_Rosa. Data from PDS Geosciences Node [7, 8].
Acknowledgements
This research has been funded by the European Research Council CoG 818602.
References
[1] Das, D. et al., 2020. Journal of Geophysical Research: Planets, 125(8), e2019JE006301.
[2] Fairén, A. G. et al., 2015. Geochemistry, Geophysics, Geosystems, 16(4), 1172-1197.
[3] von Strandmann, P. A. P. et al., 2020. Elements: An International Magazine of Mineralogy, Geochemistry, and Petrology, 16(4), 253-258.
[4] Gale, A. et al., 2013. Geochemistry, Geophysics, Geosystems, 14(3), 489-518.
[5] Parkhurst, D. L., and Appelo, C. A. J., 2013. U.S. Geological Survey Techniques and Methods, 6(A43), 497.
[6] Zhong, J. et al., 2020. J. Colloid. Interf. Sci. 572, 107–113.
[7] Wiens, R. 2021c. MSL Mars ChemCam libs spectra 4/5 RDR V1.0 [Dataset]. NASA Planetary Data System.
[8] Wiens, R. 2021d. MSL Mars ChemCam remote micro-imager camera 5 RDR V1.0 [Dataset]. NASA Planetary Data System.
How to cite: Losa-Adams, E., G. Fairén, A., and Gago-Duport, L.: Li intercalation in aluminosilicate fracture fills at Glen Rosa targets, Gale crater, Mars, Europlanet Science Congress 2024, Berlin, Germany, 8–13 Sep 2024, EPSC2024-575, https://doi.org/10.5194/epsc2024-575, 2024.
We have been conducting Earth-analog studies for Mars in Svalbard since 2008. The program includes qualitative studies of individual landforms, mapping efforts, and long-term monitoring studies. We have acquired high-resolution aerial datasets covering the years 2008, 2020, and 2024 of selected key regions in Svalbard. Data include the visual, NIR, thermal, and high-resolution DEMs. Interpretations using remote sensing have been accompanied by annual ground truth. Fieldwork involves structure-from-motion techniques, kite imagery, and measuring several weather and soil parameters during the active warm season. We will showcase some of the outcomes of previous studies and provide an outlook on the seasons to come.
How to cite: Johnsson, A., Hauber, E., Hiesinger, H., Scmedemann, N., Johantges, A., Sassenroth, C., Bucher, T., and Gessner, M.: Svalbard Permafrost Landforms as Analogues for Mars (SPLAM): Scientific outcomes and outlook , Europlanet Science Congress 2024, Berlin, Germany, 8–13 Sep 2024, EPSC2024-1092, https://doi.org/10.5194/epsc2024-1092, 2024.
Understanding the formation mechanisms of polygonal patterned grounds in hyper-arid environments like the Atacama Desert is crucial for unraveling their significance as an analogue for extraterrestrial landscapes, notably Mars. Unlike their extensively studied periglacial counterparts, the genesis of hyper-arid polygons requires more detailed exploration, since multiple processes, such as, desiccation [1] (including gypsum dehydration), thermal contraction [2], and haloturbation [3] are currently under debate. Therefore, we excavated a trench in polygonated ground in the Yungay area of the Atacama Desert and systematically collected over 80 soil samples covering an entire polygon, its adjacent sand wedges, and underlying sediments (190 x 190 cm). Sedimentological and geochemical analyses indicate a continuous interplay of atmospheric salt deposition, dissolution, precipitation, and inducing clast heave and shattering, under extreme dry conditions. Our findings emphasize that polygons in the Atacama Desert provide a promising analogue to Martian saline polygons, emphasizing the relevance of terrestrial studies for advancing our understanding of extraterrestrial landscapes.
References:
1 Ewing 2006
² Sager 2021
³ Zineabelidin 2024 (preprint)
How to cite: Anderson, J., Sager, C., Airo, A., Miedtank, A., Schwonke, F., and Feige, J.: Exploring Polygonal Patterned Grounds in the Hyper-arid Atacama Desert: Insights into Formation Mechanisms and Implications for Martian Analogues, Europlanet Science Congress 2024, Berlin, Germany, 8–13 Sep 2024, EPSC2024-1115, https://doi.org/10.5194/epsc2024-1115, 2024.
Please decide on your access
Please use the buttons below to download the supplementary material or to visit the external website where the presentation is linked. Regarding the external link, please note that Copernicus Meetings cannot accept any liability for the content and the website you will visit.
Forward to presentation link
You are going to open an external link to the presentation as indicated by the authors. Copernicus Meetings cannot accept any liability for the content and the website you will visit.
We are sorry, but presentations are only available for users who registered for the conference. Thank you.
Introduction
Mud volcanism is a widely distributed geological phenomenon on Earth1. Likewise, it has been suggested that mud volcanoes might exist on some other solid-surface bodies in the Solar System, such as Mars2 and the dwarf planet, Ceres3. Since this phenomenon requires liquid water, extraterrestrial mud-volcano-like (MVL) structures represent key targets for studying the hydrology and potential habitability of subsurface environments on other planetary bodies. Thus, identifying the potential morphological signatures of these landforms beyond Earth is an important step in understanding the nature of aqueous environments within the Solar System. Previously performed low-pressure experiments have shown that in case of “cold” surfaces (-15 °C), low viscosity mud propagates similarly to pahoehoe lava types on Earth4, while in case of “warm” (+20 °C) and unconsolidated surfaces, mud “levitates'' and hence can be transported to longer distances5. The effect of composition, however, was not further tested nor discussed. We hypothesize that the potential muds on Mars or other planetary bodies may naturally contain a certain amount of salts which can affect their antifreezing and rheological properties. Therefore we address the question how the salt component in muds may affect their propagation over cold surface in reduced atmospheric pressures, namely those valid for recent Mars.
Methods
To test our hypothesis, we carried out 54 experiments by experimental procedure adapting the settings (Fig. 1) established for mud flow experiments performed in the Large Mars Vacuum Chamber at the Open University, UK by4,5. During the experiments, portions of mud were released onto a pre-cooled sand surface when the desired pressure (5.9±1 mbar) was reached. The mud was composed of D.I. water, bentonite and with various concentrations (0.5-10%) of salts, namely NaCl, MgSO4 (epsomite), Na2SO4 and CaSO4 were tested. Experiment progress was recorded by cameras situated at the top and sides of the sandbox and temperatures of sand and mud reservoir were measured by thermocouples. In complementary methods, we investigated the pressure-drop-induced evaporative cooling of the brine component on isolated samples in low pressure. Further, we calculated theoretical p-T paths by thermodynamic modeling and measured rheological properties of salty muds for reference Earth atmospheric pressure.
Fig. 1: Setup of the (a) flow and (b) tube experiments.
Results and discussion
Our experiments confirmed expected contrasting behavior of salty mud in decreased pressure (Fig. 2). Individual flows are characterized by unique spatial dispersion and morphological patterns for mutually comparative salt content. Results also revealed thresholds when different muds produce similar patterns and spreading style for highly different concentrations. Performed brine evaporative-cooling experiments showed that the maximum antifreeze potential has NaCl and therefore solutions with this salt are capable of sustaining their liquid state in much smaller pressures than those with other tested salts. The rheological measurements, on the other hand, revealed a contrasting impact of salt addition to viscosity drop of mud samples until these are supersaturated by salt (typically >5-10% concentration in dependence on salt type) and viscosity is further increased. Due to the synergistic effect of decreased viscosity (Fig. 3a) and anti-freezing effect (Fig. 3b) flows are spatially longest for various salts and, not necessarily directly proportional, to their concentrations. For example, 2.5% NaCl mud has a higher anti-freezing effect and lower viscosity compared to 10% MgSO4 (epsomite), resulting in long and narrow flows (Fig. 3c). The increased viscosity of MgSO4 effectively slows the flow, supports the formation of a protective crust and the development of serial, long lobes that maintain liquid mud in their interiors (Fig. 2b,3c). Both salts then exhibit similar spatial dispersion but entirely different styles of propagation and surface geometric pattern. These results are contrasting to previously published experiments and reveal that the increased content of salts leads to different regimes of mud propagation, not necessarily similar to pahoehoe lavas.
Fig. 2: Plan view of representative mud flows based on various salts and their concentrations. Visible are distinct patterns of individual flows and contrasting spatial dispersion.
Fig. 3 Interpretation of the rheological and thermodynamical effects on mobility of the mud mixtures. (a) shows the progressive increase and decrease of the mud bulk shear velocity in dependence on salt content. (b) is the interpretation of how the individual salts in various concentrations (sorted from lowest to highest effect) impact the freezing delay during the pressure-temperature change. (C) three main contrasting evolutionary trends in dependence on salt type and concentration: 1. multiple short flows that form as parallel lobes, 2. single long-narrow flow - that forms as serial rope-shaped lobes for 10% MgSO4 and lobe-less gently roped pattern for 2.5 NaCl, and 3. single wide and longer "sheet-shaped" flow. The most effective propagation is observed with a combination of 10% MgSO4 and 2.5% NaCl, where the synergistic effects of minimum viscosity and lowered freezing point are most pronounced.
All these findings therefore suggest that the salt type and concentrations dissolved within the muddy mixture are important factors in controlling the ultimate shapes, textures and dynamics of mud flows emplaced e.g. on Mars or on other bodies with a thin or non-existent atmosphere. Higher salinity levels extend the unfrozen state and promote the wider spatial dispersion of muds until they reach a certain point of saturation with salts. All this suggests that on Mars, contrary to Earth, salts can play an important role in shaping MVL structures. Variations in salt types and concentrations might help to, at least partly, explain the large variability in the shapes of these hypothesized martian MVL structures.
References
[1] Mazzini & Etiope (2017). Earth-Science Reviews, 168, 81-112; [2] Brož et al. (2023). Earth Surface Dynamics, 11(4), 633-661; [3] Ruesch et al. (2019). Nature Geoscience, 12(7), 505-509; [4] Brož et al. (2020). Nature Geoscience, 13(6), 403–407; [5] Brož et al. (2020). EPSL, 545.
How to cite: Krýza, O., Brož, P., Fox-Powell, M., Pěnkavová, V., Conway, S., Mazzini, A., Hauber, E., Sylvest, M., and Patel, M.: The effect of salts on the mobility and shapes of mudflows in low pressure environments, Europlanet Science Congress 2024, Berlin, Germany, 8–13 Sep 2024, EPSC2024-1134, https://doi.org/10.5194/epsc2024-1134, 2024.
In an experiment at Martian gravity (on parabolic flights), Martian pressure, and Martian insolation we simulated the motion of particles on Martian slopes with an inclined particle bed, representing Martian soil.
The insolation generates temperature gradients within the soil which lead to thermal creep gas flow. This generates a sub-soil overpressure millimetres below the surface. The increased pressure supports particles against gravity. Here, we show for the first time that this support allows particles to move downward at much shallower slopes than on non-illuminated parts of the slopes. We also show for the first time, that on inclined slopes, the low Martian light flux is already sufficient to induce this motion (Bila et al. 2024).
While low ambient pressure is usually not in favour of particle motion as it requires high wind speeds to pick up grains, this is not the case in the presence of thermal creep gas flow. In this case, the low ambient pressure on Mars is rather a booster for particle motion. The pressure at the surface of Mars is exactly in the pressure range, where thermal creep works best (Bila et al. 2023).
At a given pressure, thermal creep depends on grain size. For soils of predominantly large sand-sized particles, no support will be present on Mars but for soils with significant fractions of smaller grains and dust, the flow features on slopes might be influenced, i.e. shaping Recurring Slope Lineae. Also, an easier downward motion of grains might increase the amount of dust that becomes entrained into the atmosphere.
- T. Bila, G. Wurm, K. Stuers, K. Joeris, and J. Teiser, Dry Downhill Particle Motion on Mars, Planetary Science Journal, (accepted), 2024.
- T. Bila, J. Kollmer, J. Teiser, and G. Wurm, Thermal Creep on Mars: Visualizing a Soil Layer under Tension, Planetary Science Journal, 4:16 1-8, 2023.
How to cite: Wurm, G., Bila, T., Stuers, K., Joeris, K., and Teiser, J.: How sunlight facilitates particle motion on Martian slopes, Europlanet Science Congress 2024, Berlin, Germany, 8–13 Sep 2024, EPSC2024-78, https://doi.org/10.5194/epsc2024-78, 2024.
Please decide on your access
Please use the buttons below to download the supplementary material or to visit the external website where the presentation is linked. Regarding the external link, please note that Copernicus Meetings cannot accept any liability for the content and the website you will visit.
Forward to presentation link
You are going to open an external link to the presentation as indicated by the authors. Copernicus Meetings cannot accept any liability for the content and the website you will visit.
We are sorry, but presentations are only available for users who registered for the conference. Thank you.
Please decide on your access
Please use the buttons below to download the supplementary material or to visit the external website where the presentation is linked. Regarding the external link, please note that Copernicus Meetings cannot accept any liability for the content and the website you will visit.
Forward to session asset
You are going to open an external link to the asset as indicated by the session. Copernicus Meetings cannot accept any liability for the content and the website you will visit.
We are sorry, but presentations are only available for users who registered for the conference. Thank you.