Introduction: Kelvin-Helmholtz instability (KHI) is considered one of the main processes of transferring solar wind energy, momentum and plasma inside magnetosphere. At the Earth, KHIs are observable in the form of waves (KHWs) within the magnetopause region between the anti-Sunward magnetosheath and the relatively stagnant magnetosphere.
Over the past few decades, several missions (THEMIS, Cluster, MMS) have contributed significantly to our understanding of KHI. In this sense, it is well-known that (i) KHIs are a common phenomenon [1,2], (ii) KHIs can be generated under different IMF conditions [2], (iii) KHIs can lead to rolled-up vortices [3], (iv) KHVs drive the onset of magnetic reconnection (Vortex-Induced Reconnection) leading to development of Tearing Mode instability [4] and formation of magnetic islands, evolving into flux ropes [5], and (v) in the late nonlinear phase, vortex merging and secondary KHIs development in a wider latitudinal range [6]. In addition, [7] found that KHWs occur at the (flank) magnetopause for approximately 19% of that time. The occurrence of these waves is influenced by factors such as solar wind speed, Alfven Mach number and number density, and is mostly independent on the IMF magnitude. These conditions can be easily met when a perturbation propagates within the interplanetary medium, such as during the occurrence of a coronal mass ejection.
Investigating the conditions under which KH and TM instabilities occur in the Earth environment, using simultaneous multipoint in-situ measurements and MHD simulations, is intriguing because it could provide insights into the flow dynamic nature at the magnetopause mixing layer. In this sense, we analyzed data from THEMIS and Cluster spacecraft considering two "target" Space Weather events occurred on 21 June 2015 (Case-1) and on 6 September 2017 (Case-2).
The Model: Our analysis utilized a 2D MHD model [8] which describes the flow dynamics of the magnetopause mixing layer in a fluid limit. The simulation domain consists of a rectangular region in (x,y)-plane. On the local Cartesian grid, it is defined as follows: (i) neglecting the realistic curvature; (ii) considering the x-coordinate pointing to the direction along the velocity of the incident magnetosheath flow; (iii) assuming the y-coordinate in the direction downward to the Earth’s center (from the magnetosheath to the magnetosphere) and (iv) ensuring a right-handed coordinate system with the z-coordinate.
The used approach is flexible enough to represent any position on the dayside magnetopause.
Results: In Case-1, we used our MHD model to interpret observational data and to investigate the potential development of KHVs on the dawn flank magnetopause as a consequence of the arrival of the ICME. Using THEMIS-E data, we found that at the magnetopause nose no rolled-up KHVs developed due to the absence of a shear between the two fluids. On the contrary, structures similar to magnetic islands appeared in By component very fast and vanished for 10 computational seconds. At the dawn flank magnetopause, the analysis of Cluster data revealed high flow and low magnetic shear between the magnetosheath and the magnetosphere. According to theoretical predictions, these conditions favour the onset of KHI. MHD simulations confirmed these considerations, finding that KHVs developed very rapidly and persisted up to 20 computational seconds (Figure 1), reaching almost MHD instability steady state. Regarding the TM instability, the MHD simulations revealed only an early development of magnetic islands (Figure 2), that persisted for half of the time of the KHVs evolution. In a global scale, these results indicate that vortices become unstable far away from the subsolar point in the direction of high flow shear. 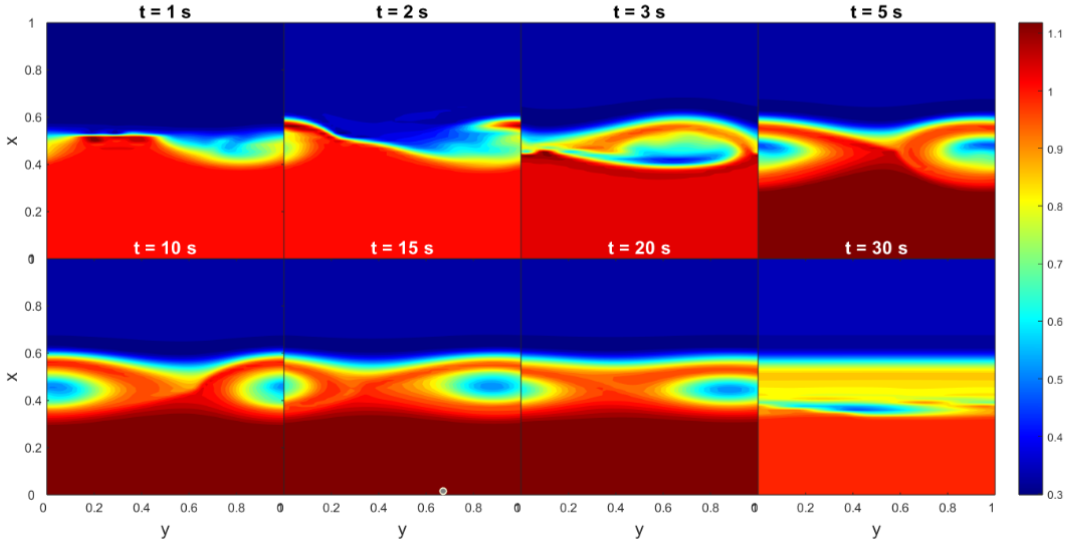
Figure 1. Case 1. Evolution of KH instabilities in density at the dawnward flank of the magnetopause, from 1 to 30 seconds. Input data obtained from the Cluster-C4 measurements. The dimensionless boundary conditions from the magnetosphere side and magnetosheath side are taken everywhere to be identical: BMSPx=-1.24, BMSHx=1.0, BMSPy=0.4, BMSHy=1, ρMSP =0.2, ρMSH=1, Re= 250 and Rm= 1 000, MA= 0.2. Blue region represents the magnetosphere whilst red region represents the magnetosheath.
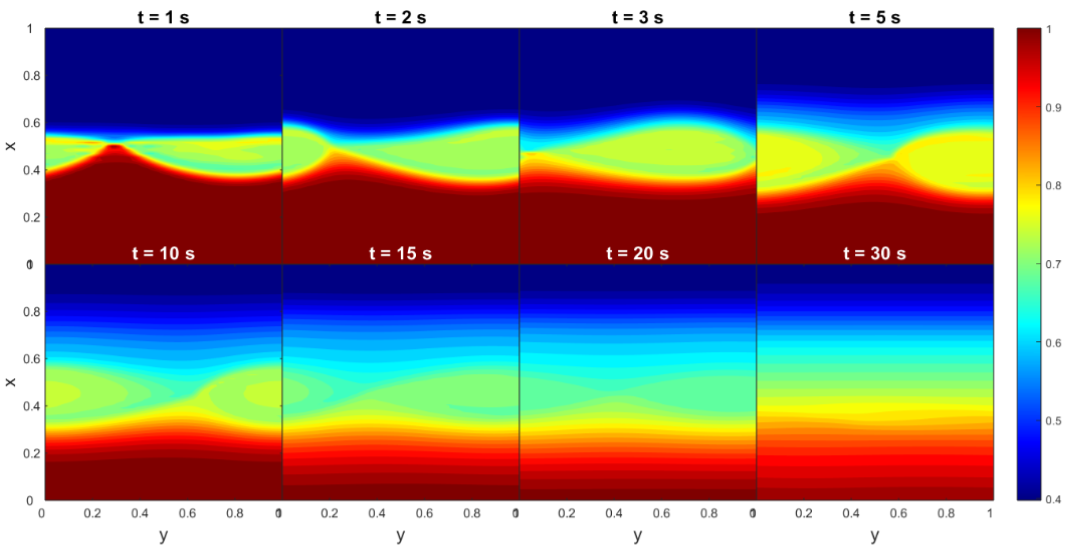
Figure 2. Case 1. Evolution of TM instabilities in By at the dawnward flank of the magnetopause. Same of Figure 1.
In Case-2, using THEMIS-E data, we did not find any evidence of KHI owing to the extremely low flow and high magnetic shear. On the contrary, adopting Cluster-C4 data, MHD simulations revealed that the fast development of disturbances but no signatures of KHVs were visible. Additionally, magnetic islands appeared very fast as a result of high shear in the components of the magnetic field but rapidly vanished.
References:
[1] Hasegawa, H., et al. (2004). Nature, 430, 755–758.
[2] Hasegawa, H., et al. (2006). JGR, 111, A09203.
[3] Lin, D., et al. (2014). JGR, 119, 7485–7494.
[4] Chen, Q., et al. (1997). JGR, 102, 151–162
[5] Eriksson, S., et al. (2009). JGR, 114, A00C17.
[6] Sisti, M., et al. (2019). Geophysical Research Letters 46, 11,597–11,605
[7] Kavosi, S. and Raeder, J. (2015). Nature Communications, 6, 7019.
[8] Ivanovski, S., et al. (2011). Journal of Theoretical and Applied Mechanics, 41, 31–42